Semiconducting layer puts out fire risk in electric vehicles
A technique to form protective semiconducting passivation layers on the surface of lithium (Li) electrodes to prevent electric vehicle (EV)-related battery fires is being put forward at the Korea Institute of Science and Technology (KIST).
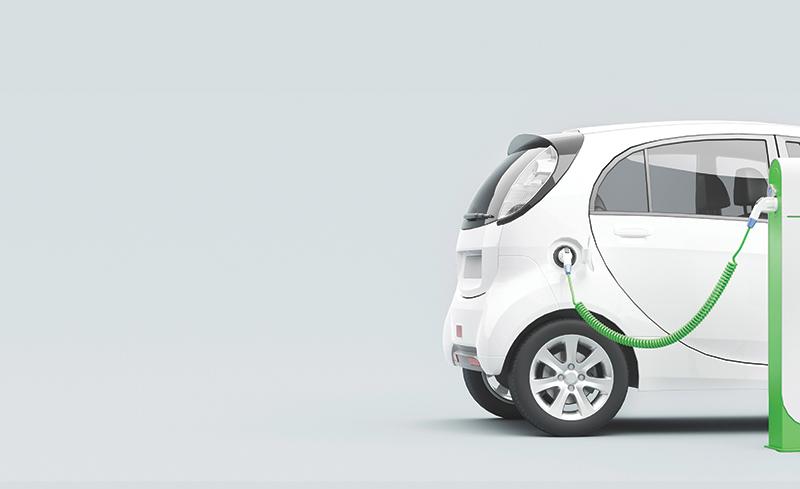
A technique to form protective semiconducting passivation layers on the surface of lithium (Li) electrodes to prevent electric vehicle (EV)-related battery fires is being put forward at the Korea Institute of Science and Technology (KIST). It works by inhibiting the growth of dendrites during the charging-discharging cycle. The team has exposed fullerene (C60) to radio-frequency plasma thermal evaporation to suppress dendrite growth. This process forms semiconducting passivation carbonaceous layers between the Li electrode and the electrolyte.
‘Fires in batteries occur when lithium dendrites continue to grow until they touch the cathode and cause a short circuit…In particular, when a piece of lithium metal separated from lithium dendrite comes into contact with air by impact in the event of a vehicle accident, spontaneous firing occurs, and an explosive fire occurs with an electrolyte composed of organic materials,’ explains Dr Joong-kee Lee, Professor at the Center for Energy Storage Research at KIST.
‘We used a C60 material precursor…to manufacture plasma polymerised carbon semiconductor thin films. We transform n-type C60 to p-type plasma carbon thin film during our study because we expect to get lower electronic conductivity and higher ionic conductivity on p-type material compared to the n-type one.’ Due to the generation of the Schottky barrier, the semiconducting passivation carbonaceous layers allow Li-ions to pass through while blocking electrons, and preventing electrons and ions from interacting on the electrode surface and inside, which stops the formation of Li crystals and the consequent growth of dendrites.
‘The electronic and ionic conductivity properties of the p-type carbon thin film also support the Schottky barrier role to prevent electrons from moving from lithium metals to the p-type carbon thin film,’ says Lee.
‘As a result of our research, when a plasma polymer carbon thin film with semiconductor properties comes into contact with the lithium metal surface, the energy bandgap of the semiconductor carbon thin film is controlled so that deposition of lithium metal can occur beneath the p-type carbon thin film. ‘Since the location where lithium ions meet electrons in the lithium electrode is fixed, dendrite generation is fundamentally prevented, and as a result, the safety of EVs using secondary lithium batteries can be ensured.’
The team has tested the electrode’s stability with the semiconducting passivation carbonaceous layers by using Li/Li symmetric cells in electrochemical environments where typical Li electrodes remain stable for up to 20 charge/discharge cycles.
‘The newly developed electrodes showed significantly enhanced stability, with Li dendrite growth suppressed for up to 1,200 cycles,’ Lee explains. ‘Moreover, using a lithium cobalt oxide (LiCoO_) cathode in addition to the developed electrode, approximately 81% of the initial battery capacity was maintained after 500 cycles, representing an improvement of approximately 60% over conventional Li electrodes.’
Lee and the team will now be investigating more commercially viable solutions. ‘We are looking for cheaper fullerene alternatives, for example. We are further investigating techniques that can control the height of the Schottky barrier, which, from a theoretical point of view, make better stabilisation of lithium metal anode during charging-discharging battery cycles.’