Photocatalytic materials in healthcare
Professor of Nanotechnology at the Clore Laboratory, University of Buckingham, Jeremy J Ramsden FIMMM, on how photocatalytic antimicrobial surface coatings can reduce infection.

Everyone agrees that hospitals should be clean, but there is no consensus on how that cleanliness should be achieved. Perhaps surprisingly — presumably because it was always taken for granted — cleaning has only recently begun to be subjected to serious scientific investigation.
In recent years, the topic has emerged as one of acute importance because the rise of antimicrobial-resistant bacteria has rendered healthcare-acquired infections extremely problematic, with a non-negligible probability of a fatal outcome, and this problem is likely to become even more severe in the next few years.
Apart from cosmetic considerations, the focus has been on the microbial burden of the environment. Cleanliness is routinely audited, but often only by visual appraisal. Measuring the microbial burden — for example as colony-forming units per cm² — is a troublesome and expensive task, even for a large hospital that will have its own microbiological laboratory.
In the NHS, wards are typically deep-cleaned with hydrogen peroxide vapour and steam once a year. The procedure is onerous, requiring patients to be decanted — not easy to arrange given that almost every bed is usually occupied. Typically, the ward has to be closed for one week to enable deep cleaning to be carried out. It is supposed to bring the entire ward environment — walls, ceilings and all fixtures including beds and bedside furniture to a sterile state, although careful investigation has revealed that the efficacy may be less than is commonly supposed. Thereafter, the ward is re-occupied and routine cleaning consists of a daily wipe with detergent. USA hospitals routinely use disinfectant, but in the UK, disinfectant would only be used if there was an unusual outbreak of a pathogen, such as norovirus. This seems to be sensible practice given that microbes can acquire resistance to disinfectants, as well as to antibiotics.
Pathogenic microbes are introduced by the patients themselves, as well as healthcare staff and visitors. Systematic encouragement of hand washing by healthcare staff goes back at least as far as the pioneering work of Ignaz Semmelweis in the middle of the 19th Century in Vienna, and although compliance is still far from complete, we have probably reached the limit of what is practical. Despite the daily cleaning, the microbial burden inexorably increases until the next deep cleaning, and there are ample studies showing how microbes present in the ward environment are acquired by patients.
The problem is, of course, that most patients have weakened or overburdened immune systems or are otherwise susceptible to infection. Yet, keeping their environment as clean as it needs to be in order to ensure the absence of nosocomial infection has remained stubbornly persistent.
As a result, there has been much recent interest in new, and not so new, technologies for keeping hospitals clean. One is ultraviolet radiation. The challenge with this is that those wavelengths that are germicidally effective are also harmful to humans, hence cannot be used in their presence. Furthermore, they generate ozone which is — although an effective microbicide in its own right — also toxic to humans. Like the hydrogen peroxide vapour used in deep cleaning, ultraviolet radiation and ozone cannot be used routinely while a hospital ward is being occupied.
It has been known for millennia that silver and copper are effective microbicides. The copper castle in Hans Christian Andersen’s tale The Tinder-Box would doubtless have been an extremely sterile place. Nevertheless, the expense and aesthetics of replicating this idea in a modern hospital make it impracticable. Furthermore, it might be too much of a good thing for the healthcare workers since these materials are also toxic and may be allergenic to humans. Some efforts have been made to use silver and copper nanoparticles by incorporating them into surface coatings but without encouraging results, not least because the coatings need to be formulated with a binder, which hinders direct contact between the metal and the microbe.
Widening the band gap
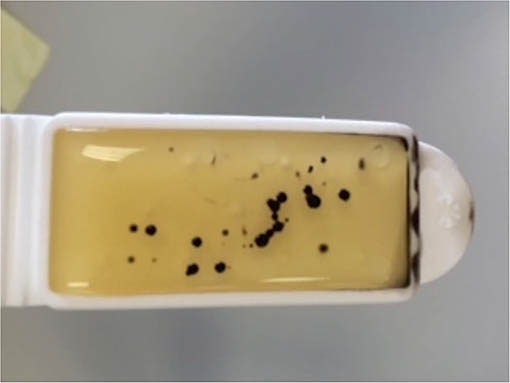
A more promising approach has been the application of photocatalytic materials. These are wide band gap semiconductors. The absorption of light causes an electron in the valence band to be excited across the band gap into the conduction band, leaving behind a defect electron – positive hole. In a normal ambient environment, oxygen and water will be adsorbed on the surface of the semiconductor, especially if it is a metal oxide, in which case the water will likely be dissociated to hydroxyl ions and protons.
The trick is to choose a material with a band gap wide enough for the electrons and holes to be, respectively, powerful reductants and antioxidants, with band edges at the appropriate levels to reduce oxygen and oxidise hydroxyl ions. If these conditions are fulfilled, one creates superoxide radicals and hydroxyl radicals. The latter is a powerful oxidant and the superoxide can pick up a proton to form an even more powerful oxidant, the perhydroxyl (hydroperoxyl) radical.
Although there are myriads of semiconductors, only a few binary oxides have been found to fulfil the above criteria, notably titanium dioxide and zinc oxide. Among the ternary oxides, strontium titanate is a good candidate – doubtless more, as well as quaternary, quinary oxides, remain to be discovered. Titanium dioxide has the important advantage of being resistant to photocorrosion, a process in which the photogenerated electrons and holes reduce and oxidise the lattice itself.
Lessons from titanium dioxide
Early interest in titanium dioxide photocatalysis was in its ability to split water into hydrogen and oxygen, for solar energy capture and storage. One important result that emerged from the work was that oxidising species can be stored for some time on the surface. The next application was the destruction of persistent organic pollutants. Then, in the mid-1980s, came the discovery of the antibacterial action of illuminated titanium dioxide.
It took many more years before this discovery was transformed into a viable technology. Titanium dioxide particles, are of course, already widely used as a white pigment in foodstuffs and paint. One is familiar with the phenomenon of chalking of outdoor paint, in which the binder is photocatalytically destroyed, allowing the titanium dioxide to be wiped off with a finger.
The challenge for an antimicrobial coating is to create a film without binder. This can be done by energetically spraying an aqueous suspension (sol) of the particles onto the substrate and allowing it to dry, during which the particles coalesce – a sol–gel process. The final result is a durable, ultra-thin film adhering to the substrate with a force comparable to the internal cohesive forces of film and substrate.
Microbes landing on the surface will be oxidised — mainly to CO₂ — provided there is sufficient water, oxygen and illumination. Oxygen is always present in abundance, and even a dry atmosphere of 10% relative humidity would provide ample water molecules. The limiting factor is usually light, but daylight entering through windows and typical indoor lighting is more than sufficient to eliminate the microbes at the rate at which they will land from an atmosphere with the usual contamination found in hospitals.
In order to generate sufficiently powerful oxidants, the band gap of the semiconductor needs to correspond to the violet end of the spectrum. Hence, only a small fraction of the light emitted from an incandescent bulb could be used. Modern lighting sources no longer emit black-body radiation and their light tends to be concentrated towards the high-energy end of the visible spectrum. Determining the action spectrum of the microbicidal effect is an onerous undertaking and does not yet appear to have been carried out.
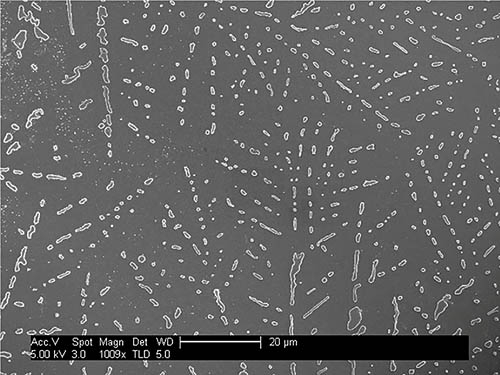
In any real material, of course, there is no abrupt absorption cut-off at the band gap because of the presence of surface states within it. The way the photocatalytic material is prepared can optimise the type and concentration of these states, which enable longer-wavelength light to be used.
A stealth mechanism
An important piece of the puzzle of the extraordinarily effective microbicidal action of illuminated titanium dioxide was only discovered last year — the stealth mechanism.
Bacteria have several antioxidation defence systems based on enzymes, although not against the perhydroxyl radical, which are triggered, for example, by strong ultraviolet radiation. The irradiance needed to photocatalytically generate the oxidising species is, however, so much weaker that it fails to trigger defence systems. Hence the oxidants can attack undetected and the bacterium succumbs.
We conclude that photocatalytic antimicrobial surface coatings offer a very promising materials-based solution to the problem of hospital hygiene and healthcare-associated infections, especially by antimicrobial-resistant bacteria. By generating extremely powerful oxidising radicals under weak illumination, these coatings kill microbes that have landed on them by a stealth mechanism, evading the cell’s antioxidation defence mechanisms. In the case of the most powerful radical generated, there is anyway no defence and appears to be no way in which the microbes could develop resistance against them — although one should never underestimate the power of evolution.
Once applied, the coating is essentially indestructible unless forcibly removed by abrasion or painted over. Being colourless and so thin as to be invisible, it is aesthetically acceptable under all circumstances. By working autonomously and continuously, it also manages to eliminate the human factor in assuring a clean environment.
For readers wondering whether photocatalytic surfaces coatings are effective against coronavirus, a virus is a smaller and simpler entity than a bacterium, but similarly constituted from oxidisable organic matter and is likewise destroyed, as has been confirmed by laboratory studies. Ensuring that surfaces of places where coronavirus might be prevalent are photocatalytically coated could well be a cost-effective method of lowering the probability of infection by eliminating viruses deposited around.