In recovery – safely removing radioactive debris
Extremely dangerous radioactive debris remains at the world’s largest nuclear disaster sites, new materials developed by scientists at the University of Sheffield, UK, could pave the way for its safe removal.
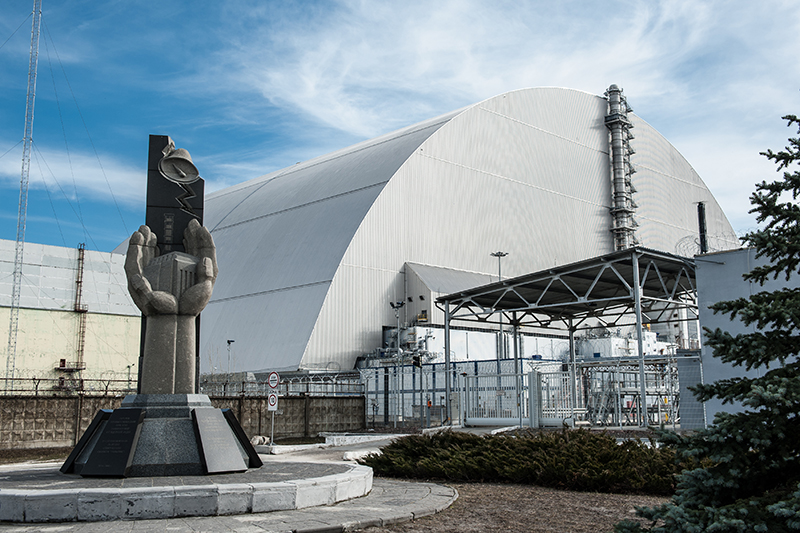
Professor Claire Corkhill, Chair in Nuclear Material Degradation, explains.
Both Chernobyl in Ukraine and Fukushima in Japan stunned the world when their nuclear power stations went into meltdown. The incidents, particularly in the case of Chernobyl, may seem long ago now, but the most dangerous radioactive materials they produced still very much remain and are slowing down efforts to clean up the sites.
During both nuclear accidents, a loss of cooling caused uranium fuel to melt together with zircaloy cladding and all the materials used to build the nuclear reactors, including concrete and steel. Due to the intense heat, the materials melted together and formed a lava-like substance that has since solidified and remains at the bottom of the stricken reactors.
In the case of Chernobyl, the mixture of molten fuel, cladding, steel and concrete, combined with sand, which was used in an attempt to extinguish the fire in the reactor at the time of the accident, formed nearly 100t of highly radioactive glass-like lava. This flowed through the nuclear power plant and has solidified into large masses such as the infamous Elephant’s Foot – a large mass of corium and other materials formed underneath the plant, composed primarily of silicon dioxide, with traces of uranium, titanium, zirconium, magnesium and graphite.
In Fukushima, the exact composition of the degraded nuclear fuel material residing in the three damaged nuclear reactors is still unknown. It is thought to comprise melted mixed oxide fuel – containing plutonium as well as uranium – zircaloy cladding, boron carbide fuel rods, stainless steel, as well as a high-sand-content concrete.
These degraded nuclear fuel substances – known at Chernobyl as Lava-like Fuel Containing Materials (LFCMs), and as Molten Core Concrete Interaction (MCCI) products at Fukushima – are highly radioactive and too dangerous for humans and even some robots to get close to, even with the most protective nuclear safety equipment. This means that only a few samples have been collected from Chernobyl, and none from Fukushima, resulting in us knowing very little about the substances and their chemical properties.
Without understanding their chemical make-up, it is challenging to design safe strategies to remove, store, or dispose of degraded nuclear fuel, and this is slowing down efforts to decommission both sites – 11 years on from Fukushima and 36 years since Chernobyl.
The degraded nuclear fuel materials present a highly dangerous risk to personnel and the environment in the surrounding areas of Ukraine and Japan. If left at the plants, they could remain a hazard for decades, even millennia, unless something can be done to stabilise or remove them.
At Fukushima, as long as the MCCI material remains, it requires continual cooling, generating millions of cubic metres of radioactive water. Controversially, this tritium-contaminated water is destined for release into the sea.
In Ukraine, there is a pressing need to remove the LFCM from the reactor building of Unit 4 before the sarcophagus, hastily-erected in the aftermath of the accident, collapses. Such an event would spread contamination throughout the reactor and significantly hamper the clean-up operation.
However, despite these huge challenges, there is hope that we can safely decommission both disaster sites, and this hope has been boosted by a major breakthrough in the world of materials science.
Safe simulation
Our team at the University of Sheffield, UK, has developed new materials that are a safe simulation of the degraded fuel materials that remain both in Chernobyl and Fukushima. These materials are a low-radioactivity version of what we think the fuel debris is like, which can now be used to learn more about their chemical properties and behaviour so we can begin to design safe strategies to remove the real degraded fuel.
The research has been led by academics in our Department of Materials Science and Engineering at Sheffield, using the HADES and PLEIADES National Nuclear User Facilities, and is providing unique insight into these complex and fascinating materials.
Using this simulant material, we have already identified the potential whereabouts of plutonium in the debris at Fukushima for the first time – a major step that can help inform the design of removal strategies. For Chernobyl, the simulant material has started to help with understanding the corrosion mechanism of LFCMs, which creates hazardous radioactive dust inside the reactor building.
Honing the recipe
To make this breakthrough, our team took inspiration from the only available degraded fuel materials, recovered from the Chernobyl reactor in 1991. The bulk composition of these materials was used to design a recipe for the simulants, and, with some trial and error, a melting process was developed to closely replicate them.
Importantly, the simulants omit the most radioactive portion of the fuel – the fission products – which only account for a small fraction of the chemical composition, but most of the radioactivity.
The melting conditions have been fine-tuned so that the resulting simulant material – a highly heterogeneous glass ceramic – contains the same uranium and zirconium-containing crystalline phases as present in the real LFCM. This includes the rare mineral chernobylite – a high uranium-content zircon (Zr1-xUxSiO4, where x < 0.10) not found in nature. Not only were we able to achieve the same phases, but their distribution in the glassy matrix are also very similar.
Tiny fragments of the simulant LFCM, no larger than 1mm, were analysed by micro-focus X-ray spectroscopy and diffraction techniques at the National Synchrotron Light Source-II in the USA and at the Swiss Light Source. Here, we were able to understand uranium’s chemistry in the crystalline phases and, for the first time, in the glass, which differs depending on whether the LFCM is iron-rich – representative of LFCM containing a high steel content – or not.
The LFCM simulants are currently undergoing a two-year corrosion test at the UK Diamond Light Source’s unique, long-duration, X-ray diffraction facility. Here, we are learning more about the formation and nature of uranium-containing corrosion products, such as thermonatrite (Na₃H(CO₃)₂.₂H₂O).
Such phases formed when ‘rain’ fell on the LFCM – due to water condensing on the inside of the roof of the sarcophagus, dripping onto the reactor and degraded fuel below. These corrosion products are extremely dusty and present a respirable hazard to workers during decommissioning operations.
Replicating conditions
With a verified methodology to replicate LFCM from Chernobyl, the same approach was used to replicate MCCI from Fukushima. Although we do not yet know the precise composition of the degraded fuel at this location, we have worked closely with our collaborators at the Japan Atomic Energy Agency to use the most accurate recipe for the simulants.
One of the main differences between the Chernobyl and Fukushima degraded fuels is the presence of plutonium in the latter, due to the use of mixed oxide fuel in one of the reactors. Since plutonium is a fissile material and can undergo spontaneous nuclear fission, understanding its location is of utmost importance in the decommissioning operation. In our simulation, we used the element cerium as a surrogate for plutonium, since it carries many similar chemical properties.
The simulant Fukushima MCCI materials were found to contain many of the same phases as the Chernobyl LFCM, including uranium and zirconium oxide minerals distributed within a glassy matrix. Since it had a much higher silica content than the Chernobyl LFCM – due to the use of a high-sand-content concrete at Fukushima – there were many more silicate minerals present, including those that incorporate the plutonium surrogate, cerium.
In particular, cerium is found to partition into the glassy matrix and also into the mineral phase perclevite (Ce₂Si₂O₇). The proximity of the cerium in the simulant MCCI to iron, from the melting of steel, also changes its oxidation state, which may have implications for plutonium’s stability during corrosion of MCCI by cooling water in the reactors.
Finally, working with our project partners at The University of Bristol, UK, the simulant materials from both reactors have been analysed to estimate their mechanical properties using high-resolution synchrotron X-ray tomography at the Diamond Light Source. This was coupled with digital volume correlation analysis revealing that both materials are brittle, and the Young’s modulus and Poisson ratio can be determined. Ultimately, this information can be used to help design the cutting tools on robots to remove the fuel material from the reactors.
Clean-up
In summary, our research has proven that the rich chemical and mechanical property information about degraded nuclear fuel materials from accident scenarios can be derived from small fragments of low-radioactivity simulants.
Since reducing the size of highly radioactive materials also reduces their hazard, it is hoped that once the first fragment of real MCCI is recovered from Fukushima, the same characterisation techniques can be applied. By learning more about the nature of the fuel, the decommissioning process could be accelerated and these hazardous reactors could be cleaned up, once and for all.
Chernobyl's impact
It is estimated that all the xenon gas, about half of the iodine and caesium, and at least 5% of the remaining radioactive material in the Chernobyl 4 reactor core (which had 192t of fuel) was released in the accident.
Most of the released material was deposited close by as dust and debris, but the lighter material was carried by wind over Ukraine, Belarus, Russia and Scandinavia.