High-energy hopes at the European XFEL
The European XFEL research hub in Germany hosts the world’s largest X-ray laser. Alex Brinded catches up with Dr Sakura Pascarelli, one of three Scientific Directors.
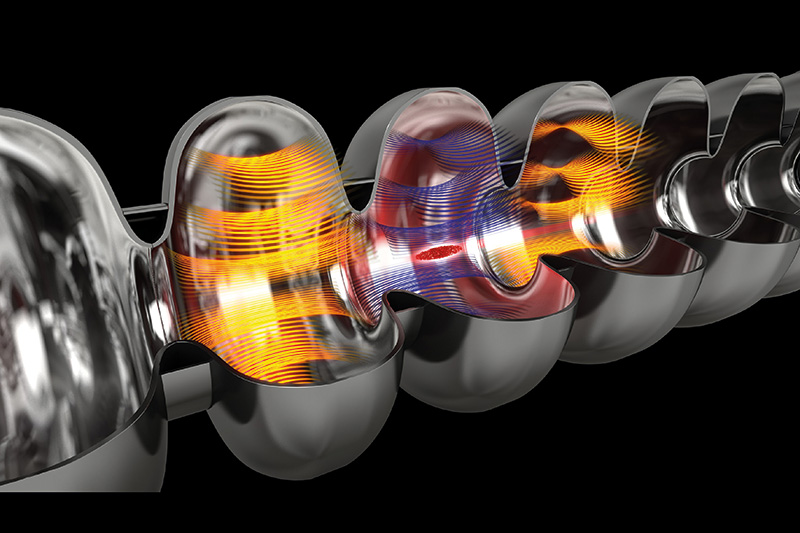
To generate the short, fast, intense X-ray flashes at the European XFEL in Germany, bunches of electrons are first accelerated to high energies and then directed through undulators that contain special arrangements of magnets. Radiation is then emitted in laser-like flashes.
The first part of the expansive facility is a 1.7km-long particle accelerator that brings the electrons to high energies, where they travel at nearly the speed of light.
The electrons are generated in the injector in an underground building on the Deutsches Elektronen-Synchrotron (DESY) Campus. They are then accelerated in niobium-based superconducting resonators. In these, an oscillating microwave transfers its energy to the electrons. The resonators are cooled to a temperature of -271oC when they lose their electrical resistance, and nearly the entire electrical power is transferred to the particles.
The accelerator delivers compact and ultra-short electron bunches, a prerequisite to operate an X-ray laser.
The accelerated electrons then race through the arrangements of magnetic undulators, which force the particles onto a tight slalom course, and each individual electron emits X-ray radiation that is then amplified.
The European XFEL has three such long undulators of more than 100m. Each undulator can provide a beam to two to three instruments that are located in a large experimental hall under the European XFEL administration building on the Schenefeld Campus, at approximately 3.4km from the injector.
Dr Sakura Pascarelli, one of three Scientific Directors at the facility, describes how the electrons wiggle and emit radiation at every bend, which is travelling tangentially to the electron’s trajectory. By making the turns shallow, she shares how the X-rays cause greater intensity and brilliance.
As the radiation is faster than the electrons speeding along their slalom path, the radiation overtakes the electrons flying ahead and interacts with them along the way, accelerating some of them and slowing others down. As a result, the electrons within a given bunch gradually organise themselves into a multitude of thin disks, from which they emit their light in sync. This results in extremely short and intense X-ray flashes with the properties of laser light.
Pascarelli points out that ‘synchrotron’ is actually the name of the electromagnetic radiation emitted when relativistic charged particles are subject to an acceleration perpendicular to their velocity. When the velocity of the particle is very high, like these electrons, it can be collimated, collected and sampled.
At the European XFEL, a sophisticated electron-bunch distribution system distributes bunches into each undulator individually, thus enabling the simultaneous operation of several experiments.
The European XFEL for now has three undulators with six experiment stations, with plans to expand to five undulators with 10 instruments, perhaps more. Today, four of these instruments use ‘hard’ X-rays (typically between 5,000-25,000eV) and two use ‘soft’ X-rays (typically between 300-3,000eV).
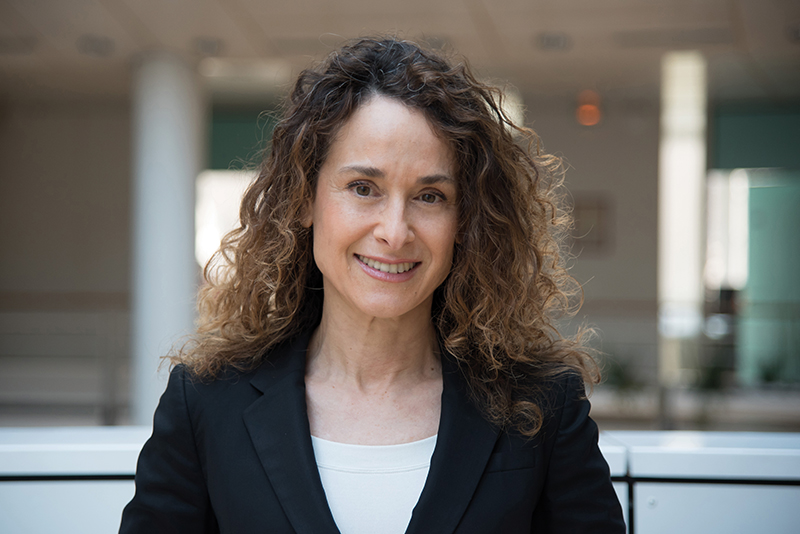
New worlds
The facility is reportedly making previously inaccessible research possible. The aim is to use the X-ray flashes to map the atomic details of viruses, decipher the molecular composition of cells, take 3D images of the nanoworld, film chemical reactions, and study processes such as those occurring deep inside planets.
Depending on the experimental requirements, the flashes can be widened, focused, filtered, or weakened using optical elements such as mirrors, gratings, slits, or crystals. The interactions between the samples and the flashes are measured and recorded using special detectors.
Pascarelli and her fellow Directors in the Management Board are responsible for the independent peer review of all research activities to be performed by users at the facility. External experts meet regularly to review and rank submitted proposals by scientific relevance. Although she has no say in this ranking, she seeks to assure the integrity of the decision-making.
'I try to make sure that the best science is done on the instruments,' she asserts. After being presented with the rankings, she is responsible for the final selection of experiments to be scheduled on the four hard X-ray instruments. Since three instruments run simultaneously and require different characteristics, working out the common accelerator parameters that will benefit all experiments, and primarily the electron energy required, is not trivial.
'Making the schedule is a nightmare,' she admits, but fortunately she has the support of her fellow Scientific Director.
Did you know?
- The European XFEL based near Hamburg, Germany, is located mainly in underground tunnels that can be accessed from three different locations.
- It generates ultra-short X-ray flashes of 27,000 times per second, with a duration of less than 100 quadrillionth of a second (less than 100 femtoseconds).
- The flashes have a brilliance billions of billions times higher than the best conventional X-ray sources, and a billion times higher than modern storage-ring-based X-ray sources.
- The facility aims to map the atomic details of viruses, decipher the molecular composition of cells, take 3D images of the nanoworld, film chemical reactions and study processes that occur deep inside planets.
- The 3.4km-long research centre comprises tunnels that run from the German national research centre – Deutsches Elektronen-Synchrotron (DESY) – in Hamburg to the nearby town of Schenfeld in Germany.
Imaging the atom
The first undulator to be commissioned at XFEL was the SASE 1 in 2017. It is the radiation source for two instruments that Pascarelli is responsible for – the Femtosecond X-ray Experiments (FXE) and the Single Particles, Clusters, and Biomolecules & Serial Femtosecond Crystallography (SPB/SFX).
The FXE carries out time-resolved investigations of the dynamics of solids and liquids, while the SPB/SFX instrument achieves ultrafast diffraction and coherent imaging of single particles, clusters and biomolecules.
Pascarelli says that using coherent imaging to see the 3D structure of a single protein molecule at the atomic scale has been 'one of the dreams for X-ray free lasers”. She notes that great progress has been achieved in this field at other XFEL facilities, and more recently at this site – today they can deliver atomic resolution for strong scatterer particles like gold, but for weak scatterers such as proteins, it is still a long and winding road.
'We’re not there yet, nobody is able to do this because it is much more difficult than one thought. It’s really the background signal that is limiting us for these kinds of measurements. We can do single-particle imaging at an XFEL, but not on proteins and not the atomic scale, not with angstrom resolution, but let’s say with few nanometres resolution. So that’s the challenge...'
The SPB/SFX beamline also does 'very interesting megahertz microscopy experiments' to image fast processes. Pascarelli suggests this has applications for fundamental materials science, but also for welding and additive manufacturing, because movies can be made with an image every microsecond and spatial resolution close to the micrometre.
Pascarelli shares, 'At the European XFFEL, we have a peculiar time structure of the pulses that come in trains.' There is a train of pulses every 100 milliseconds, with a pulse every 200 nanoseconds within those trains. 'So, if you use these trains of pulses, you can capture a movie,' including of irreversible processes, because the intensity of each pulse is so high that a single one generates an image with sufficient signal to noise ratio.
Pascarelli foresees great potential for materials research, especially if the energy of the X-rays is ramped up from 20-25KeV to 50KeV+ in the future. They are exploring the opportunities of very hard photon energy to 50KeV and beyond.
The FXE can be applied to all materials, she says, including energy materials such as chemical complexes for photocatalysts, to understand what happens to the electrons that are generated through photo-absorption, and why the charge is lost through vibrations and heat.
'If you could take the charge generated by the photoabsorption process and transfer it efficiently to the active catalytic site of the molecular complex, which does the job of, let’s say, splitting the water molecule to create hydrogen, then it would become an efficient process. Today we are not able to do it. So, the idea is to understand why,' says Pascarelli.
X-ray vision
Soft X-rays carry far less energy than hard X-rays and are, for this reason, more easily absorbed by air and other mediums. In water, the majority of soft X-ray photons will be absorbed before they have travelled even a millionth of a metre, while hard X-rays can be used to look at broken bones or to investigate the atomic-level properties of solid materials.
Source: www.anl.gov/article/7-things-you-may-not-know-about-xrays
Ramping up the intensity
While the two instruments on SASE 3, the soft X-ray undulator, are not her responsibility, Pascarelli notes that it is placed right after SASE 1, which means that the electrons first travel through SASE 1 before they enter into SASE 3. The staff of the two beamlines therefore need to closely collaborate to make sure that their respective experiments run smoothly.
The SASE 2 undulator, meanwhile, produces hard X-rays for the High Energy Density (HED) instrument and the Materials Imaging & Dynamics (MID) instrument. The HED investigates matter under extreme conditions using hard X-ray Free-Electron Laser radiation, e.g. probing dense plasmas, while the MID determines the structure of nanomaterials and dynamics at the nanoscale using X-ray photo correlation spectroscopy. With this method, Pascarelli says MID is currently investigating dense, antibody drugs.
The researchers compare the changes that occur across separate pulses for many different timescales. However, in the early days, they were concerned about particle damage because the X-rays are so intense. 'We were scared that you would actually cook your protein, you would change it,' says Pascarelli. 'This is what was happening at the beginning,' but according to Pascarelli, they now better understand this phenomenon and can control it.
Discussing the HED instrument, Pascarelli describes how it comprises two very high-powered lasers, one being a femtosecond laser, which deposits 10J of energy in 10 femtoseconds – 'huge, huge powers. Hundreds of terawatts on the sample'.
Or they can use a long pulse laser, with pulse duration in the nanoseconds, 'with a lot of energy, like 100J. When this laser hits the sample, it ablates the surface and generates a shock wave that travels at the speed of sound through the remaining part of the sample. It is this shock wave that compresses and heats the sample to huge pressures and temperatures'. This enables probing of the structure at extremely high pressures.
HED also employs diamond anvil cells to compress the sample between two diamonds to a certain pressure. Then the very intense X-ray pulses to heat the sample, while simultaneously revealing the structure from the diffraction signal. 'This is all new stuff that we are starting now,' adds Pascarelli.
Life in the fast lane
While Pascarelli was new to what she calls this 'ultra-fast science', on joining in 2019, she brought much experience to the role from her 30-year career with hard X-ray synchrotron radiation.
After finishing secondary school, Pascarelli studied physics at La Sapienza University of Rome, Italy, and, in 1990, was offered a scholarship at the National Institute for Nuclear Physics in Frascati, just outside Rome.
In those years, the high-energy laboratory was being used 'parasitically' by material scientists and physicists who were using the radiation produced by the accelerated electrons. 'These were the first-generation machines, so we were using synchrotron radiation in a parasitic way. Typically, only when the particle physicists allowed us to use it,' Pascarelli says.
The team Pascarelli was part of was preparing the design for the Italian beamline at the European Synchrotron Radiation Facility (ESRF), known as the General-purpose Italian Line for Diffraction and Absorption (GILDA).
When the ESRF storage ring in Grenoble, France, completed construction and was ready to install beamlines in 1993, Pascarelli moved there to assist with building the instrument she had helped design. 'When we started building it, somebody had to go and live there permanently, and nobody wanted to go in my group – everybody had a family. I was the youngest in the group.'
And although she was not responsible for the project, with the other technicians, engineers and scientists staying for weeks or so at a time, she became de facto in charge of GILDA as the permanent fixture. 'Every single problem that there was, I had to solve. And I think that was a very, very important part of my career where I learned a lot.
'Coming from a small team, I was in this huge international centre. I had to communicate with people. Of course, I had a lot of help from the ESRF technical staff, but the collaborating research groups are supported mainly by national laboratories and this staff was not there all the time.'
She continues, 'It was a difficult period, but that’s when you really learn. We were encountering a lot of phenomena with these new beams that nobody could really explain.'
After the ESRF was inaugurated in 1994, GILDA was opened along with other beamlines.
Ring in the changes
From parasitic radiation use in the first-generation synchrotrons, the second-generation machines were rings built specifically for the purpose of solid-state physics, chemistry, material science and biology. Meanwhile, the third generation were designed as high-brilliance sources exploiting undulators in long, straight sections of the storage ring.
She shares how the third-generation sources produced X-rays with high transverse coherence, as in opitcal lasers, which changed the way the images were analysed. All beamlines had windows because everything was in a vacuum, and they would observe interference effects on the images, as every tiny imperfection on the window became a small source of X-rays, 'and nobody understood what they were'.
Now coherence is used for imaging. Coherent imaging is one of the most important techniques that has been developed at synchrotrons, with totally new applications from paleontology to archeology, and from medicine to cultural heritage, but this was unknown before the ESRF became operational.
Pascarelli points to this as an example of the difficulty scientists have of explaining how they will use a tool before it exists. 'Because we don’t necessarily know what science we’re going to do. You have to make the tool, and then you discover new phenomena that allow you to think in a different way, do new things that you don’t even know how to explain…It’s very interesting.
'I’ve lived through this, so I know what I’m talking about. But it’s funny how every time you have to do an upgrade, the funding bodies want you to justify it.'
She says that the ESRF was the first truly high-energy storage ring because the electrons are accelerated to 6GeV, when the radiation becomes 'extremely collimated, almost parallel'.
Following on from ESRF, other high-energy synchrotrons built around the world further increased their energies, including the Advanced Photon Source (APS) at the Argonne National Laboratory in Chicago, USA, at 7GeV and SPring-8 in Japan at 8GeV. This led to larger rings, with more space for beamlines and also higher photon energies.
But one of the most important figures of merit for storage rings is brilliance, or photon density on the sample, which scales inversely with the so-called ‘emittance’ – a measure of the electron beam size and divergence.
Pascarelli recalls that until about 15 years ago people thought that an existing storage ring could not be upgraded to deliver X-rays with a considerably higher brilliance, while maintaining constant other key parameters such as electron beam energy, current, lifetime, source stability and operational reliability.
The ESRF Machine Director at the time, however, had a 'very brilliant idea” and designed a revolutionary storage ring, which has a different layout of the magnets fitting in the existing infrastructure and enabling even smaller emittances. That was an important step for the migration of existing storage rings towards fourth-generation synchrotrons.
The ESRF indeed has just been upgraded accordingly to be the ESRF/EBS, which stands for Extremely Brilliant Source. 'The ESRF was the first of the high-energy synchrotrons to do this transformation,' says Pascarelli. 'It started from there and now everybody’s doing it. And apparently 6GeV seems to be the optimal energy.'
Pascarelli says that the APS and SPring-8 are now lowering back down to 6Gev. 'The PETRA III facility in Hamburg is also going to upgrade – they are waiting for funding.'
Deep focus
Pascarelli spent 26 years at the ESRF. 'I’ve lived through the whole evolution of these synchrotrons.'
She was first recruited as the scientist in charge of the ESRF’s beamline ID-24 in 1997 – an undulator beamline for X-ray absorption spectroscopy. 'This was mainly devoted to time-resolved applications, looking at chemical reactions…I transformed it to also perform high-pressure science because that was what I was interested in.'
Pascarelli was invested in magnetism and in particular in ferromagnetic metals at the time. 'It’s really interesting to know what happens to iron when you compress it because the centre of our planet is made predominantly of iron, in the solid core where it is subject to millions of atmospheres – when you compress iron, it starts losing its ferromagnetism and actually becomes non-magnetic at a certain pressure.' After starting with iron, she looked at cobalt, nickel and their alloys.
At that time, Pascarelli was the only woman in charge of an instrument at ESRF, let alone two. In 2000, she also became responsible for Beamline BM-23, leading a large group of technicians scientists and postdoctoral fellows.
'Things are changing now, but at the time, it was not very easy to get women into this business. I never saw it as a difficulty, actually I was very proud of being an example for young girls. I never felt that I had a problem being the only woman.'
Like her work, Pascarelli approaches her hobbies with the same drive and commitment. “I am a very focused person, but I also always stress the importance of having a balanced life. So, for example, I wanted to have a family, I wanted to have a healthy life, I always wanted to do sports', with swimming her major sport at a competitive level.
X marks the spot
At the European XFEL, Pascarelli is now the Scientific Director of four large groups, while at the ESRF, beyond her administrative duties as Group Head, she was scientist in charge of beamlines, and 'very much hands-on'.
She reflects, 'There was actually no reason for me to leave the ESRF – it was a fantastic, sort of dreamlike environment…But either I stayed there or moved onto the European XFEL, where I can learn new things, because an XFEL opens the window into ultra-fast science', because the pulses are at femtoseconds.
'I knew I could be useful because they need X-ray expertise here. But I also knew that I was coming in with zero knowledge in ultra-fast science.
'A lot of the ultra-fast science that has been performed for decades using optical lasers is now being transferred to X-ray Free Electron Lasers, with the main advantage being much shorter wavelength. We are trying to learn from the laser scientists, the methods they used and apply them to the XFEL.'
Pascarelli clearly has a zeal for where she works, as well as for the science and techniques. 'Given my background, I grew up in Asia and I came back to Europe when I was 16 – for me, Europe is like a unique nation. The European XFEL is really international, with staff from 60 different countries. I love being in an international environment.'