Fusing foundations - the potential for industrial symbiosis
The UK Research and Innovation Foundation Industries Future Leaders Group explores how there are increased opportunities to share resources to move towards an industrial circular economy.
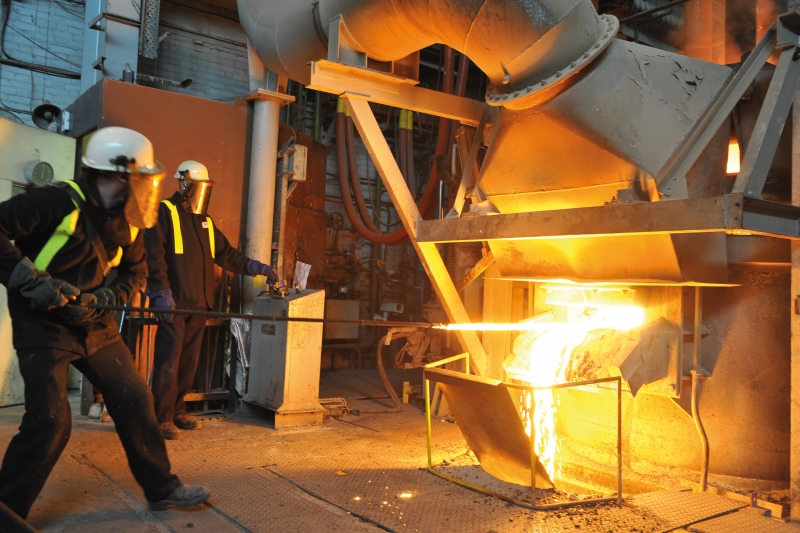
The Foundation Industries (FIs) encompass the metals, ceramics, glass, chemicals, paper and cement industries in the UK. Together, these industries produce nearly 30Mt of material annually and play a critical role in our everyday lives – 75% of the materials we see around us are made by the FIs.
This large-scale production contributes to a combined total of approximately 50Mt of CO₂e emissions per year, which accounts for ~10% of the UK’s total emissions.
The Innovate UK Transforming Foundation Industries Challenge Fund has been running since 2020, and has encouraged these six industries to work together to disrupt existing supply chains, share skills and experience, and develop innovation to reduce their environmental impact.
In this article, we highlight how industrial symbiosis (the sharing of resources between industries to promote a circular economy) is already being used, and the areas in which more work needs to be done to ‘close the loop’. It should be noted that the examples provided reflect mainly the knowledge of the authors and may not be exhaustive.
Cement
Cement plant, solid-material, waste generation is relatively low compared to other FIs. For example, much of the dust and other by-products are returned to the kiln or blended in the final cement in diluted quantities. But this is not the full story, large amounts of gaseous ‘waste’ material are produced, i.e. CO₂.
In the UK, cement is used in three main ways – ready-mixed concrete (55%), pre-cast products (23%) and builders’ merchants (19%). Collectively, UK manufacturers have the capacity to supply up to 10Mt of cement clinker per annum – the amount of cement will be greater depending on blending (clinker factor) of cements – which is a significant source of CO₂ emissions.
During the production of clinker, the raw meal loses ~40% of its weight, which is mostly CO₂, not considering the CO₂ released during fossil fuel combustion to achieve the necessary high temperatures for clinker production. Converting this CO₂ from a liability to an asset (such as minerals or fuels) could be crucial in achieving sustainability in the cement industry.
One major solid waste stream that is generated in specific modern plants is cement kiln bypass dust, which contains significant quantities of salt, such as potassium chloride. It is technologically possible to return this material to the process. However the main barrier to doing so is the cost of removing the soluble salt, whether via dissolution or thermal evaporation.
Modern cement plants are also energy efficient. For example, the major heat output stream (flue gas) is normally used to preheat raw materials, and energy from the combustion gases is mostly recovered due to the counter current (heat exchange) nature of kiln configurations. In fact, modern kilns are more energy efficient than F1 race cars. But there is still room for improvement.
When air is used as the oxidant during the combustion of fuel, energy is used to heat up nitrogen gas from the air and is not fully recovered.
Oxyfuel combustion and/or electrification of kilns can therefore result in improved energy performance.
Overall, the cement industry is a net consumer of solid waste during production, but there is room for further incorporation. The industry benefits from not having a problem of demand compared to other commodities that have lower requirements. To support an increasing population and urbanisation, cement is required for housing and infrastructure with no other material that can completely replace the quantities required. Cement therefore has a key role in any foundation industry symbiosis because there is opportunity to accept foreign solid and gas material or energy streams.
One of the most significant waste streams from the construction industry is demolition waste, which can be fully recycled, albeit currently only in an open-loop manner. The recycling of demolition waste will need material input as it is not as effective as it could be. This also offers opportunities for the cement and concrete sector. With the development of advanced separation and grinding technologies, there is the opportunity for closed-loop recycling of cement by refiring the hydrated cement material back into clinker, while recycling both the coarse and fine aggregate back directly as aggregate.
Meanwhile, bulk solid waste streams from other FIs contain the main elements required for cement production – calcium, silicon, aluminium and iron. Re-processing these materials in the right quantities should be able to produce a reactive clinker or mineral additive chemistry. However, understanding the effect of unconventional ‘minor elements’ or contamination (such as heavy metals) is a major challenge.
For the cement industry, where there are various stakeholders who need to approve any changes to specification, it is not a trivial exercise to introduce foreign elements into our building stock without proving completely that there will be no adverse effects – people’s lives are literally at stake. Proposing new cement products is therefore a painful process because society trusts the material it has been using and developing for the past 200 years – Portland cement. Any change suggested will be extremely slow and incremental because the industry is resistant to change.
The position of active quarries is also an area of concern. The cement industry needs to start looking into positive contributions to biodiversity through ongoing site management and wildlife-focused restoration schemes, especially when looking into new ways to produce cementitious materials. Already excavated and landfilled materials should be considered for further processing to establish and adopt circularity within the construction sector.
Iron and steel
Iron and steelmaking results in the production of by-products in the form of slags, sludges and dusts. Although some of these by-products are still landfilled, many have been a perfect example of industrial symbiosis for over a century.
For example, blast furnace slag and basic oxygen steelmaking slag (BOS) has been used in the construction industry since 1865. BOS slags are commonly used as aggregates and in road construction. Recent developments in this area have seen steelmaking slags being used to improve the wear and skid resistance of asphalt.
More recently, research efforts are on the use of electric arc furnace (EAF) and basic oxygen furnace slags as cement precursors and replacements in clinker production.
Blast furnaces slags (produced during the ironmaking process) are also split into two categories: air-cooled slag and granulated slag. Air-cooled slags are used within the aggregate and road construction industry, although research is ongoing to expand this use to other industries. Granulated slag is mainly used in the cement industry where it is processed further to ground granulated blast furnace slag (GGBS).
GGBS is used throughout the world as a Portland cement replacement. The benefits include improved durability and CO₂ reduction with up to 90% material replacement achieved depending on the application. Other uses for granulated slag are within the glass industry as the raw material calumite. In the glass industry, the benefits of using slag include savings in energy, CO₂ reduction and improvements in product quality through defect reduction and redox control.
The sludges produced in a blast furnace during ironmaking are not yet reused. Due to their high zinc content, it is difficult for them to be recycled back into the blast furnace, but they also contain a high iron content and therefore are prime examples of how further research is required to support a circular economy.
The UK’s Materials Processing Institute is working with the domestic iron and steelmaking sector to determine the most efficient, environmentally friendly and economically viable recovery method for these materials. The Department of Materials Science and Engineering at the University of Sheffield, UK, is investigating using these side-streams for clinker production.
Aluminium
The production of primary aluminium from bauxite ore results in the formation of bauxite residue. Significant quantities of this by-product are produced with an estimated 160Mt in 2022 alone. This is predicted to increase to more than 220Mt by 2050 with the increased demand for aluminium across most sectors.
Bauxite residue is alkaline in nature and therefore requires careful and rigorous management to avoid catastrophic environmental and social incidents. This can occur from leaching or spillage in the local community. Currently, the predominant method of management is to store the bauxite residue in designated bauxite residue storage facilities. Even though these facilities are carefully managed, this practice does not promote a circular economy, particularly as the residue can contain other valuable metallic materials beneficial to other FIs.
One of the key high-impact opportunities for the use of bauxite residue is in the cement and concrete industries. Bauxite residue can be used in the production of cement clinker, cement precursor, and supplementary cementitious material for blended cements. The implementation of bauxite residue in these products reduces energy consumption and CO₂ emissions of the clinker/cement production, storage requirements for the residue, and natural resource utilisation. These residues can also be used to produce alkali-activated cements. Of course, barriers still exist to increasing the use of bauxite residue across the cement industry, including disseminating potential uses of the residue to companies.
Did you know?
Around 3Mt of bauxite residue are already used in the production of clinker, sourced from sites found in Greece, India, China and other countries around the world.
The UK does not produce virgin bauxite ore for primary aluminium production, but some bauxite is imported and processed in Ireland. However, legacy landfill of bauxite residue does exist and can be reclaimed.
The UK industry also produces tens of thousands of tonnes of alumina-containing side-streams from secondary aluminium production and recycling, which has little or no commercial value and are generally landfilled at a monetary cost that is carried over to the aluminium metal industry.
Hundreds of thousands of tonnes of these by-products in landfill are in the UK, and they can be reclaimed. Through UK Research and Innovation Future Leaders Fellowship research, Dr Theodore Hanein MIMMM, at the University of Sheffield, UK, is developing the use of these side-streams combined with excavation of waste clays. The aim is to establish supply chains for the production of low-carbon, high early strength (rapid set), calcium-sulfoaluminate-based cements. This is another example of national investment into industrial symbiosis in the FIs.
Future footprint
There are numerous challenges across the FIs to reduce the environmental footprint of the products manufactured. Some of these challenges apply universally across the industries, such as the requirement to decarbonise energy sources whether electrical, thermal or otherwise – and capacity for renewable energies will be in demand. This is one area in which the FIs can work together and may find additional symbiosis.
From the industry perspective, the cement and concrete sectors are historically conservative industries for several reasons. Any change not only requires innovative ideas and materials, but significant investments. But the cement industry has the lowest margin to deal with abatement, as they produce ~9kg of CO₂/£ turnover, quite high if compared to iron and steel ~2kg CO₂/£ turnover.
On the other hand, there are debates over the carbon counting procedure of materials such as GGBS and the monies received by the iron and steel sector for these materials. This further strengthens the argument that industrial symbiosis across these sectors can be extremely beneficial to all stakeholders.
The main challenges the value chain is facing and demanding from the cement and concrete sector are:
- Amendments of standards and codes to work on optimised designs which allows different materials to be used in specific applications for specific purposes (e.g. service-life requirements, minimum cement contents, water-cement ratios, etc.).
- Establishing harmonised approach towards sustainability credentials (Life Cycle Assessments (LCA), credible CO₂e data, comparable data among the industry, etc.).
- Service-life demands and end-of-life planning (or design-to-recycle)
- Updated and reliable test methods for service life and recyclability
Any integration of mass or energy cross-sector, will require physical proximity or requirement for transport over long distances. One consideration could be to locate new FI facilities in the same area so they can be more easily connected. It will not be easy or economical to transport bulk materials or low-grade heat.
While the integration of these and other examples are key drivers to addressing the net-zero challenge, it is crucial that materials and processes are considered from a whole lifecycle perspective. All the FIs use LCA as a tool for understanding the environmental footprint of the sector products and processes, generally following international standards. However, as multiple methods are relevant for by-products, each FI uses varied approaches for their own system and agenda.
As by-products could increasingly be used across the FIs, there is an opportunity to understand and harmonise environmental assessment standards. One important example of this is the carbon impact of GGBS or pulverised fuel ash, which is currently calculated based on its mass, while its economic impact is considerably higher and not considered in the UK.
Furthermore, the carbon impact of these materials and processes alone is not enough, an LCA that considers a full suite of environmental impacts including emissions to water and soil, land use, resource consumption and other impacts must be determined.
Overall, to achieve transparent and accurate assessments, data must become increasingly available to assist in robust comparisons of existing and new sustainable opportunities.
Along with the discussed challenges, all FIs have other areas for impact assessment, including social and economic impacts of these changes, in line with the UN’s SDGs. The FIs must also consider if a second life is sufficient for the products used. When developing new materials from recovered sources, we must aim to ensure that these materials in turn are recoverable to reuse and recycle.