Acoustics in action – designing in sound control
Dr Han Zhang MIMMM, Senior Lecturer in Materials Science at Queen Mary University of London, UK, discusses the design strategies in sound absorbing materials.
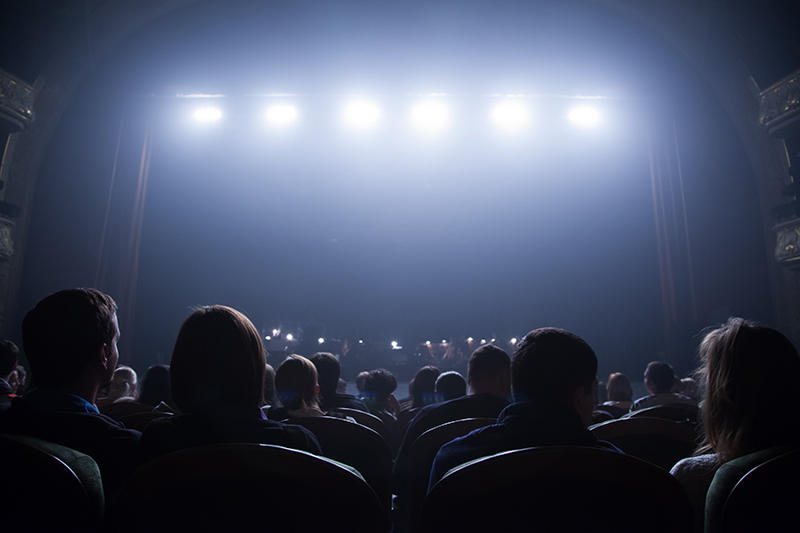
Acoustic materials are everywhere in our daily life, from sound absorbing materials in our cars to improve interior sound quality, to acoustic panels on the walls and ceilings of theatres and cinemas to prevent resonance and unwanted echoes and noises.
In fact, sound absorbing materials are helping us on an important but often overlooked mission, preventing a series of health issues such as hearing loss, stress, poor concentration, sleeping disorders and cardiovascular diseases which can be caused by exposure to noise pollution and excessive noise.
Although the terms ‘sound absorption’ and ‘sound insulation’ are occasionally used interchangeably, they are two very different concepts. Sound absorbing materials are designed to improve sound quality and prevent the aforementioned echoes and noise, while sound insulation or soundproofing acts as an acoustic barrier to block sound waves.
Among various sound absorbing materials, two common categories can be found – one is a porous-type, such as polyurethane (PU) foams and non-woven fabrics, while the second is a resonator-type, such as microperforated panels and metamaterials.
Different sound absorption mechanisms are involved with these. The porous materials exploit the frictional heating between the vibrating air molecules and pore surfaces to dissipate the sound energy, while the resonator-type materials comprise artificial periodic structures with zero or negative refractive index to manipulate and control the sound waves.
Design in sound absorption
Design strategy plays an extremely important role in both types of materials. Various design parameters can be tuned to achieve the desired acoustic performance, ranging from cell density, cell morphology, and open cell content in sound absorbing foams, to fibre types, size, shapes, fabric density and porosity in fabric-based sound absorbing materials.
Polyurethane foams and panels
With its lightweight and good damping properties, alongside a wide frequency absorption range, PU foam material is one of the most widely used sound absorbing materials.
By tuning the pore sizes of interconnected porous structures, the sound waves can travel into the foams or panels and be reflected multiple times within the pore, absorbing the energy and hence reducing the reflection back to the environment.
Although more interfaces are welcome to promote this absorption process, the pore size should not be too small as it may reduce the amount of energy entering the structures hence affecting the sound attenuation.
Foam is a typical example of a sound absorbing material with relatively easy processing and low costs, but performance over the low frequency range (100-800Hz) is often limited.
The acoustic performance, as well as the structural properties of PU foams, can be improved by adding various fillers – carbon nanofillers, rubber micro-particles and inorganic particles – or other polymers. The introduction of an appropriate amount of filler can tune the foam’s cell size and density, at the same time creating additional interfaces between the fillers and matrix to increase the structural tortuosity and energy dissipation.
Hierarchical and hybrid cellular foam structures consisting of nano-, micro-, and macro-pores have also been developed in recent years, with tailored cell morphology to achieve desired sound absorbing performance.
Commercial foams can also be tuned to achieve hierarchical pore structures via methods like freeze-drying, 3D printing, electrospinning or chemical vapour deposition, in combination with aligned nanofillers or ordered nanostructures to enhance their sound energy attenuation performance.
Textile-based materials
Textiles-based sound absorbing materials, whether based on synthetic fibres or natural fibres, are also widely used as lightweight and cost-effective sound absorbers.
Depending on the service environments, inorganic and metallic fibres can provide resistance to high temperature, flame and corrosion, while natural fibres like hemp and kenaf offer many advantages of environmental sustainability and biodegradability.
Apart from the bulk density, thickness and porosities of the fabrics, the cross-sectional shapes and fibre diameters will also affect sound absorption. For example, the use of nanofibre layers can enhance performance over low frequency range.
Textiles consisting of hybrid fibre assemblies – either different fibre materials or different fibre diameters – can also tailor their sound absorption performance at desired frequency range, fulfilling various requirements for practical applications.
Non-woven fabrics are often used in the form of pads attached to structural components, or as the surface layer of sandwich structures. By combining non-woven fabrics as sandwich skin layers with PU foams as the core, the sound waves need to travel through various paths with increased energy dissipation. Both the properties of fabric layers and foam core, alongside the interfaces between skin and core, could affect the sound attenuation.
Thermoplastic foams
Thermoplastic foams offer the benefits of recyclability and decent mechanical performance over traditional PU foams or textiles.
Microcellular injection moulding is one of the most widely used processes to manufacture thermoplastic foam sound absorbing materials. Both chemical blowing agents, which decompose during processing to generate gas, and physical blowing agents, like compressed inert gases, are used to produce thermoplastic foams.
It is worth mentioning that without the mould opening during the microcellular injection moulding process, only closed cell structures can be achieved, which only provide a similar level of sound absorbing performance as their solid counterpart.
Therefore, a secondary mould expansion step is needed to achieve open cell structures. A gas counterpressure during the mould opening process can facilitate cell uniformity and surface appearance, as well as subsequent sound absorbing performance. Like PU foams, the low frequency sound performance of thermoplastic foams is also relatively poor.
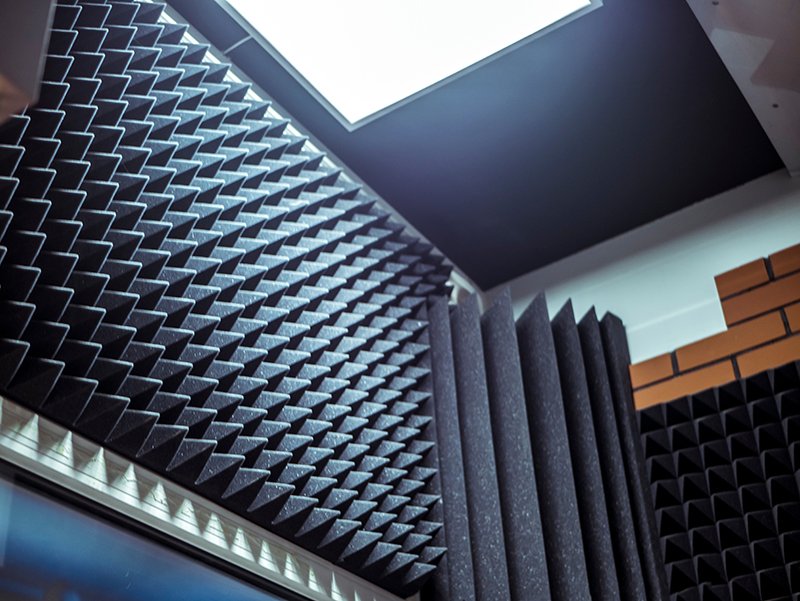
Acoustic foam on the wall of a music studio
© Mike_shots/ShutterstockMicroperforated panels and metamaterials
Microperforated panels (MPP) can be regarded as a special category of porous materials, with sub-millimetre to millimetre diameter holes in the panel surface. By adjusting patterns and parameters – like hole diameters, shape of cavity, thickness, and perforation ratio – tailored sound absorption performance can be achieved for the intended frequency range.
Honeycomb backing and partitions in the backing air cavity can improve the MPP sound absorber performance through a local 1D sound field within each air cavity subdivision.
As mentioned earlier, acoustic metamaterials, which are composed of artificially designed sub-wavelength structures, either in periodic arrangement or in specific design patterns, can achieve some unusual and extraordinary sound absorbing performance that traditional acoustic materials cannot.
By creating and arranging patterns and structures in sub-wavelength scale, local resonators can be assembled to absorb the acoustic waves. One such property – rainbow-trapping – is the ability to absorb sound perfectly and simultaneously over a wide range of frequencies. It involves incorporating or fashioning substructures at or below the wavelengths to be absorbed, refracted, or reflected.
Applications of acoustic metamaterials include seismic wave reflection and vibration control technologies related to earthquakes, as well as precision sensing. They also have a variety of engineering applications, for example, as a mechanical component in optomechanical systems.
As the designed artificial structures do not exist in nature, zero or negative refractive index can be achieved, with matched impedance to the background media. For low frequency range of 100-1,000Hz, metamaterials can demonstrate excellent sound absorbing performance, far beyond the limit of traditional acoustic materials.
Although acoustic metamaterials open up many new possibilities in sound absorbing material designs, most suffer from very narrow working frequency band, not to mention their incompatibility with traditional manufacturing methods. Specific design and integrated structure with other acoustic materials, and the use of additive manufacturing methods, can mitigate some of these challenges in applications.
From the road to home
From the padding in vehicle floor panels and door trims in our cars, to ceilings, floors and office acoustic screens between each desk in the interior of buildings, acoustic materials can be found almost everywhere in our daily life.
In the automotive sector, sound absorption materials in the form of non-woven fabrics are widely used in various locations, ranging from carpets, door trims, seat covers, to air engine bonnets and wheel liners. Thermoplastic foams with open cell or semi-open cell structures are also gaining an increased amount of attention in passenger cars for cabin noise attenuation, thanks to their ease of recycling and environmental sustainability over traditional acoustic foams.
Both foams and microperforated panels – and many times, the combination of two – can be found as the sound absorbing materials in the interior of buildings. By optimising the design of these materials, from foam structures to the shape of the acoustic foams, tailored sound absorption characteristics can be achieved to fulfil the requirements of differing environments, whether this is for offices, theatres, or swimming pools.
Another important application area is infrastructure and construction, where an excessive amount of noise can be expected from motorway, rail and air traffic. Noise panels for motorway applications often consist of both sound absorption and sound insulation designs and materials, to reduce and prevent the noise experienced by surrounding neighbourhoods as well as the drivers.
Future credentials
Although versatile design and materials solutions can be found to achieve desired sound absorbing performance, few challenges remain for the clean and sustainable growth of this field. Not surprisingly, traditional acoustic materials like PU foams are mostly landfilled or incinerated. Some very encouraging initiatives have already looked at alternative acoustic materials, for instance, using waste cotton, tealeaf fibre, or fibre fluff waste from shredded end-of-life rubber tyres as raw materials for acoustic absorption.
More importantly, a full lifecycle, rather than the usage stage only, should be considered and designed for the next- generation of acoustic materials.
From the development of new manufacturing processes with high energy efficiency and cost effectiveness, the selection of raw materials with low carbon footprint, to the end-of-life options with reuse and reprocessing enabled, a closed loop of this sector should be borne in mind when designing and making next-generation sound absorbing materials.
For more information on this topic readers can see the review paper (10.1016/j.apmt.2021.101141), or contact Dr Han Zhang directly at [email protected]
Scientists create mechanism to control sound waves in metamaterials
Physicists at University of Oregon, USA, have developed a new method to manipulate sound – stop it, reverse it, store it and even use it later – in metamaterials.
The discovery was made using theoretical and computational analysis of the mechanical vibrations of thin elastic plates. The physicists, Pragalv Karki and Jayson Paulose, also developed a simpler minimal model consisting of springs and masses demonstrating the same signal manipulation ability.
The interplay between bending stiffness and global tension – two physical parameters governing sound transmission in thin plates – is at the heart of their signal-manipulation mechanism. While bending stiffness is a material property, global tension is an externally controllable parameter in their system.
Karki and Paulose describe their new mechanism, which they call ‘dynamic dispersion tuning’, in a paper published online in the journal Physical Review Applied.
'If you throw a stone onto a pond, you see the ripples,' Karki explains, 'But what if you threw the stone and instead of seeing ripples propagating outward, you just see the displacement of the water going up and down at the point of impact? That's similar to what happens in our system.'
Optical or photonic metamaterials, which exhibit properties such as a negative refractive index not possible with conventional materials, were initially developed to control light in ways that could be used to create invisibility cloaks and super lenses.
Their use is being explored in applications such as aerospace and defense, consumer electronics, medical devices and energy harvesting.
Acoustic metamaterials are usually static and unchangeable once produced, and dynamically tuning their properties is an ongoing challenge. While the mechanism in the new paper was identified theoretically and needs to be proven in lab experiments, Karki says he is confident the approach will work.
'Our mechanism of dynamic dispersion tuning is independent of whether you are using acoustic, light or electronic waves,' Karki says. 'This opens up the possibility of manipulating signals in photonic and electronic systems as well.'
Possibilities include improved acoustic signal processing and computation. Designing acoustic metamaterials based on graphene could lead to variety of uses like wave-based computing, micromechanical transistors and logic devices, waveguides and ultra-sensitive sensors.
'Our design could be built at the microscale with graphene and at large scales using drum-like membrane sheets,' Karki says. 'You strike the chain of drums, creating a particular pattern of sound that moves in one direction, but by tuning the tension of the drums, we can stop the sound and store it for future use. It can be reversed or manipulated into any number of other patterns.'