Transforming foundation industries
With an introduction from Professor Ian M Reaney FIMMM, Director of the Transforming Foundation Industries Network+, three key projects funded by the programme are described.
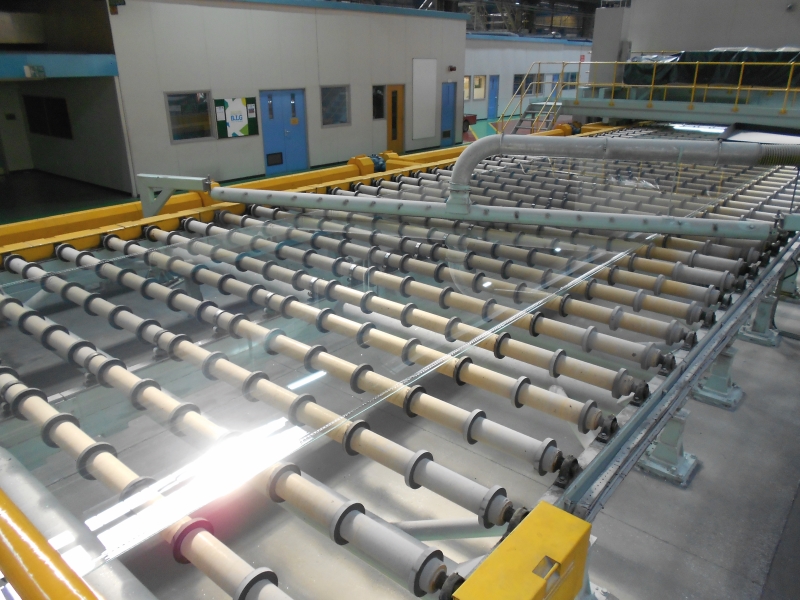
The built environment is our adaptation of the natural environment. It is complex with a multitude of materials and systems required to create sustainable cities and towns. Construction techniques and the design of buildings has improved dramatically in the last few decades, with greater emphasis placed on optimising materials use, reducing heat loss and distributing/generating heat efficiently.
However, focusing purely on improving the construction of a building does not address the ‘elephant in the room’, which is that glass, ceramics, metals and cements are produced from broadly the same energy-intensive methods used for the last 50 years. Glass, ceramics, steels and cements, along with chemicals and paper, constitute the Foundation Industries (FIs). They are the largest consumers of natural resources and industrial polluters, generating 10% of all the UK’s CO2 emissions.
The challenge to transform the FIs through resource/energy efficiency and fuel switching away from a reliance on fossil fuels is well-recognised and is being addressed at multiple levels. These efforts until recently were focused on individual sectors. However, many of the FIs face the same challenges, such as reducing/removing their reliance on fossil fuels, the need for innovation in reducing energy usage, and a growing demand to encourage greater inter- and intra-sector industrial symbiosis to minimise waste that often ends up in landfill.
Did you know?
The Foundation Industries comprise over 7,000 businesses, more than 250,000 employees, and a combined turnover of greater than £67.5 bln in the UK.
Source: Innovation Readiness in UK Foundation Industries, an Enterprise Research Centre report for UK Research and Innovation
In 2020, the Transforming Foundation Industries (TFI) Challenge was created, which uses a mixture of government and leverage funds to holistically address the issues faced by these energy-intensive industries.
The TFI Challenge is composed of key elements, each designed to address innovation in FIs, such as the Transfire Research Hub based in Cranfield, the Glass Futures Project in St Helens and the Transforming Foundation Industries Network+ (TFIN+).
The TFIN+ is based at the University of Sheffield, UK, with spokes to the Universities of Leeds, Manchester and Swansea. Its remit is to engage with the wider community of academics and industrialists who work with and within the FIs through focused workshops and seminars to curate a sense of community and promote innovation.
Its broader remit is to encourage companies who do not traditionally engage in government-funded initiatives to collaborate, stimulate interest in FI-based research among early-career researchers, and promote equality, diversity and inclusion.
A major part of the TFIN+ activities is to allocate project funds of ~£1.4mln to enable the development of small projects for the benefit of the FIs through responsive calls on key topics, including: energy efficiency, resource efficiency and industrial symbiosis, and digitisation and next-generation processes. Among these small projects, around £800k has been awarded to early-career researchers (ECRs), defined as either current post-doctoral researchers or newly appointed lecturers.
To date, 34 small projects have been funded that have led to key outputs in important areas of innovation within the FIs. All projects are led by academics (dominantly ECRs) with significant participation of industrial partners, including in-kind support.
At the time of writing, not all projects are complete, but it is evident that significant innovations have already been achieved, and this article summarises key developments to date from TFIN+ funded projects on resource efficiency, energy efficiency and digitisation. Each project addresses a key aspect of the production of lower-carbon construction materials in the FIs.
These are only three of many ambitious innovations achieved through funded research via the TFIN+. The TFIN+ has a unique role in developing a sustainable built environment through its ability to create and fund partnerships between academic and industry, encouraging engagement of ECRs, and accelerating widespread uptake of innovative, low-carbon technologies for the manufacture of construction materials.
Resource efficiency and symbiosis
Sustainable Investment Assurance Model (SIAM), Dr Stephen Spooner, Coventry University (formerly University of Swansea), UKThe project extends multifaceted sustainability modelling from the steel industry to other FIs. Sustainable Investment Assurance Model (SIAM) is an example of a unified tool used to improve decision making for industrial symbiosis and has created impact at government and industry levels. Innovation in energy recovery is vital as we move to an era in which energy is no longer an abundant cheap resource, and the use
of decision-making algorithms within glass manufacture, for example, offer the potential to optimise production yield and minimise plant downtime.
Consultation with each sector helped review the existing SIAM framework and adapt measurements concerning circularity, direct/indirect energy consumption maps, economic drivers versus policy constraints, and proportional representation of workers, working conditions and wealth disparity. The primary outcomes are summarised:
- Decarbonisation/sustainability improvements from environmental perspectives are significantly skewed to the use of additional energy requirements for either heat, transport, or reduction of material. The UK has a relatively well-developed renewables portfolio on the grid, however, over short-time scales, an increase in energy demand can only be met through the use of non-renewable energy production methods. Consequently, until further development of a renewable energy grid with overcapacity is achieved, there is minimal gain to be made from altering production methodologies.
- Volatility in supply chains is a major factor that can affect every aspect of sustainability. Without control and consistent supply of materials, validation and representation of sustainability credentials is not only meaningless but potentially unethical to provide to consumers as a purchasing motive. Supply chain volatility is a big risk factor under current global influences, such as the COVID-19 pandemic recovery, Brexit, war in developed nations and the forecast recession. These factors create an economic reluctance to both invest from an initial financial basis, but also from a point to minimise further disruption from current manufacturing methodologies. De-risking supply is another overarching theme that must be tackled to shift to sustainable practices.
- Data quality continues to be an issue. SIAM developed databases for each case study, mined from literature which required significant time and expertise. Known data was inputted into databases when available, but over 75% was obtained from open-source unverified literature. Such data collection is time consuming and expensive, but with investment in capital expenditure necessary across multiple industries to meet sustainability targets, it is essential. If integrated from the outset, data collection can bring significant improvements with minimal cost and impact.
Current discussions are ongoing with several companies on how SIAM can be used to quantify sustainability improvements of future capital expenditure investments. A limited version of the framework has been supplied to government organisations for internal use on investment decision making.
The tool is also being openly used within several large companies to validate sustainability investment plans both in the UK and international operations. The benefit of SIAM to the built environment is to facilitate informed decision making among stakeholders such as the FI, national and local government, and construction companies in their drive to reduce the carbon footprint.Energy efficiency
Investigation of a flat heat pipe for high-temperature waste heat recovery from metal slabs, Professor Hussam Jouhara, Brunel University, UK
Energy efficiency is among the most important keys to unlock a green and more sustainable economy, and a substantial amount of energy can be saved via the reuse of waste heat. Exploitation of waste heat streams has already been investigated mainly relying on forced or natural convection as a heat transfer method. However, waste heat is also available as a radiative source.
Radiative heat sources pose real challenges due to the complexity of modelling, their intensity and the status of current technology. Indeed, no technology can currently effectively recover radiative waste heat sources.
Brunel has developed a novel, radiative, flat heat pipe (FHP) heat recovery system to capture high-temperature waste heat from slags and slabs. The FHP relies on
two-phase transfer to passively move heat from the hot to the condenser section. The heat is then transferred safely to the heat sink such as air, water and water-glycol.
The FHP system was designed according to a thermal/electrical analogy, was constructed with the support of Econotherm Limited, UK, and prepared for testing. Testing was carried out in an industrial environment and, for a heat source of 450°C, the FHP was able to recover up to 800W/m² with an average evaporator surface temperature of 45°C, highlighting its potential for even higher temperatures.
The results of this industrial test, along with validation of the FHP model, has allowed Brunel to assess further use. It has been concluded that the FHP technology could be easily implemented in the steel industry, for example, in the manufacture of wire that is extruded and cooled down by forced convection and radiation on long conveyors. If applied to the entirety of the conveyor, the expected output from the FHP system is predicted to be 12.5kW/m².
The aim is to further develop the technology to full demonstration scale, initially in the metals sector, which presents strong potential for FHPs due to the high temperature and available heat sinks – but also in cements.
The built environment relies upon the metal and cement sectors for much of its materials. Heat recovery in these energy-intensive industries is of vital importance if we are to continue to manufacture in the UK. This is true irrespective of the introduction of low-carbon or carbon-neutral energy production in the future.
Digitisation
Improved energy efficiency of float glass production, Dr Peter Green, University of Liverpool, UKOf all the ‘enablers’ of transformation, digitisation and control offer the greatest potential to impact multiple FI sectors, and the benefits they bring of reduced plant downtime and energy and raw material savings are clear.
Implementation, however, deviates significantly from the classic skillsets associated with the FIs, requiring knowledge of advanced computing, sensing, modelling and data handling.
In the built environment, glass is pivotal in modern construction with 10Mt of float glass produced in Europe every year – the UK currently holds a 6% share
of European glass production.
Pilkington United Kingdom Limited, part of NSG Group, is a leading UK glass manufacturer and supplier with around 3,000 staff across the UK. It produces a
wide range of glass solutions for architectural applications, solar panels, automotive equipment, touchscreens, etc. Being an energy-intensive manufacturing process and noting NSG Group’s aim to achieve a 30% reduction in CO₂ emissions by 2030, this project explored a data-based model that can ultimately be used to increase the efficiency of float glass production.
The study involved the development and deployment of a machine learning model. It was trained on data gathered from hundreds of sensors at the UK5 float glass plant in St. Helens, UK, and was used to predict variations in product quality up to 72h into the future.
The overall model was formulated using a Gaussian ‘product of experts’ approach, whereby separate models are each used to cover different regions of the input space before predictions are formulated using a weighted product of all models.
The model is able to function in an online setting allowing it to be updated using the most recent data from the UK5 plant. The model can be used to form different decision-support tools (e.g. the optimiser, sensitivity analysis) to help increase the efficiency of float glass production. The approach is scalable to large datasets and, to the best of our knowledge, generic in that it can be extended to other float glass furnaces.
NSG has directly funded another six months of research which has already been transferred into the Manufacturing Execution System used at the UK5 plant.
Ian M. Reaney, Hussam Jouhara, Stephen Spooner and Peter Green acknowledge the support of the Engineering and Physical Sciences Research Council Grant No EP/V026402/1, Transforming the Foundation Industries (TFI): a Network+ Towards Value by Innovation. Ian M. Reaney is grateful for critical reviews of the article from Susan Bernal-Lopez, William Sampson and Cameron Pleydell-Pearce. Ian M. Reaney also thanks Bruce Adderley, Ben Walsh and Sarah Connolly from the UK Research and Innovation, Transforming Foundation Industries Challenge for their support.