Shooting for the stars - could space colonisation become a reality?
Uncovering some of the recent space technologies taking us to new heights.
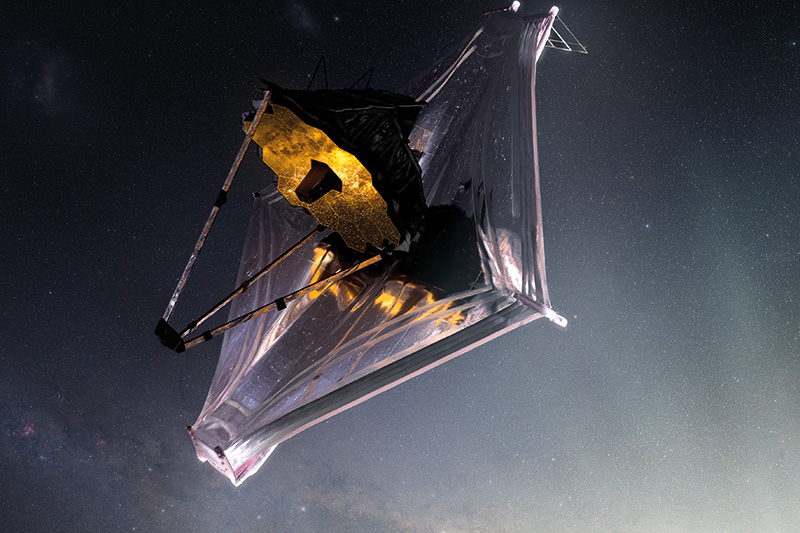
Space research is one of the most expansive fields of study in every which way. According to data provider Statista, between 2010 and 2019, global government investment in Space exploration amounted to US$167bln, and it is expected to rise further to over US$250bln in the next decade.
Beyond exploring what’s ‘out there’, research in Space also improves life on Earth. The European Space Agency (ESA) reports that studies of life and physical sciences in Space are well-established fields that complement terrestrial research programmes. Biology, physiology, fluid physics and combustion, materials science, fundamental physics, and astrobiology are all advanced when delving into Space.
Spaceflight is a driving force, for example, in developing advanced medical instruments for monitoring and diagnostics.
The ESA also reports that ‘studies in weightlessness can reveal properties that are important for energy production or environmental protection. Space research has already increased knowledge on combustion, liquids in porous substances and how dust particles behave.
‘These studies are expected to lead to low-pollution, high-efficiency combustion for power plants, aircraft and cars, as well as improved crude oil recovery, and innovative air and water purification techniques.’
Here, we look at Space research projects that have soaring ambitions.
To the Moon and back
At Sandia National Laboratories, USA, researchers are working on a resilient microgrid to sustain astronauts, mining and fuel processing on the Moon.
This project goes hand-in-hand with NASA’s concept for an Artemis lunar base that will serve as a technology-proving ground for eventual human exploration of Mars.
The base camp concept consists of a habitation unit – complete with room for up to four astronauts – as well as separate mining and fuel processing, called in situ resource utilisation facilities.
These would produce rocket fuel, water, oxygen and other materials needed for extended exploration of the lunar surface, while decreasing supply needs from Earth. It would be located far from base camp, but the electrical grids for the two units will be connected during emergencies for resiliency and robustness.
Rachid Darbali-Zamora, one of the engineers developing the microgrid at Sandia, explains,’Microgrids are smaller power grids that have the ability to separate themselves from the main power. They can independently manage and distribute local generation. They also incorporate renewable energy such as solar panels.
‘The objectives of the project are to bring adequate power management and to ensure that power is readily available for the lunar habitat, the mining and the production microgrids. This is achieved by coordinating the generation, storage and load consumption of all three of these microgrids.’
‘There are some very important differences between something like an ISS-type [isolated] microgrid to something that has the extent of a Moon base,’ continues Jack Flicker, a Sandia Electrical Engineer.
‘One of those differences is the geographic size, which can be problematic, especially when running at low DC voltages. Another is that when you start to extend these systems, there will be a lot more power electronics, as well as a lot more distributed energy resources that will exist throughout the base. Sandia has been looking at microgrids with a lot of distributed energy resources for quite a long time.’
Software will regulate the electricity used by the mining and processing centre through a controller, similar to the cruise control in a standard automotive.
The controller needs to maintain an even voltage level on several different timescales, from less than a thousandth of a second to seasons. At the highest level of the control software, on the scale of minutes to seasons, people can control which solar panels generate power and what power-using devices are turned on. However, at the lowest level, at less than a thousandth of a second, the controller needs to operate rapidly and automatically to maintain outputs at the required levels.
‘We have a specialised Secure Scalable Microgrid facility and control-system-design methodology that analyses this. The facility also has specialised energy storage emulators that can help us determine the specifications for how much energy storage the base needs and their requirements,’ shares Control Engineer Dave Wilson.
The Secure Scalable Microgrid testbed is a unique Sandia research facility to fine-tune the control system.
Questions about power system controllers and the interactions between distributed energy resources, energy storage and power electronics on a DC microgrid are also at the forefront of the investigations at the site, with a scaled and simplified representation of the eventual lunar microgrid.
The testbed consists of three interconnected DC microgrids with custom-built electronics to mimic different power-production systems and devices that use electricity, including diesel generators, photovoltaic arrays, energy storage emulators, and power converters.
Each of the emulators can be controlled by a computer, and the microgrids can be configured to test an endless variety of scenarios.
Staying connected
The researchers explain that there are two primary ways to get resiliency into a microgrid. One is to flexibly route power where it is needed. The other is to over-size everything to ensure there is enough power even if multiple things fail.
‘Usually, we have some combination of those two,’ Flicker says. ‘In a contingency event, such as an energy storage system failing during an eclipse, we want to be able to port the power at the mining facility over to the base camp to keep astronauts safe.’
Flicker’s part of the team is also exploring how the connection between the two microgrids could operate.
They are studying the impact of the distance between the two units on transfer efficiency and stability, whether they are five miles apart or 20.
The team is also determining the optimal voltage the connection should operate at, and whether it makes sense to keep it DC, or convert to AC to make the trip, and then back to DC on reaching the habitation unit.
‘With this DC power-hardware-in-the-loop set-up that we’re building in the lab, we can test power converters, the impedance of electrical lines between lunar facilities, [and] actual energy generation and storage devices,’ Darbali-Zamora adds.
‘Basically, we can use it to study a variety of situations, so we can design a system that is self-sustaining and can continue operating even if a solar panel array goes down.’
The project is currently in the design stage, but some experiments have been performed on components at Sandia National Laboratories.
Live off the land
While Sandia’s project focuses on energy for exploration and mining, HELIOS’ vision is to make humans multi-planetary and extend colonisation to the Moon and Mars.
As launchers are constantly improving and landers are nearly operational, HELIOS is developing technologies that can use resources onsite – first to drastically reduce the cost of initial establishment, and ultimately to enable the colonists to ‘live off the land’ and minimise resources taken from Earth.
The firm notes, ‘The cost of launching the required materials to set the first lunar base is currently unfeasible, only utilising resources onsite can enable this endeavour.’
HELIOS is developing a reactor that can process lunar soil and Martian soil to oxygen and metals.
CEO Jonathan Geifman explains that the Molten Regolith Reactor is intended for ‘production of oxygen on the lunar ground, to provide a propellant oxidiser, which is going to be nearly 70% of the total mass required in Space’.
It works by melting and electrolysing the regolith to separate the oxygen from the lunar minerals, producing metals as by-products.
Geifman says, ‘The concept of oxide electrolysis is not a new one – the challenge in doing it on the Moon or Mars is the need to be independent of Earth-brought consumables and high-system longevity.
‘We developed new electrodes and a reactor body that can sustain the process for long periods of times with no consumables.’
One of the advantages of using electrolysis, Geifman suggests, is that by applying specific potentials on the cell, you can control the oxides separated from oxygen in the melt.
The technology is now under development and there are plans to scale it up for testing on the Moon by the end of 2024.
The programme instills hope to eventually fabricate parts on the Moon, ‘most likely through casting, extrusion and spool making for future additive manufacturing’, Geifman explains.
Assembling the future
How will we build the coming generations of Space architecture – the modules, spaceships and space stations that will ensconce our Space-faring species? Can we move beyond the 20th Century paradigm of cylindrical tubes in orbit, to geodesic dome habitats, microgravity concert halls, or space cathedrals?
Researchers from the MIT Media Lab believe the future of human habitation in Space lies in self-assembling, adaptive and reconfigurable structures, rather than transporting fixed, rigid habitation modules and risking astronaut extravehicular activities (EVAs) during construction. Research could soon lower payload weight, reduce assembly complexity, and revolutionise space-structure modularity by relying on reconfigurable, self-assembly.
‘We are currently undertaking a multi-year research effort to study, characterise, prototype and test ‘TESSERAE’ – Tessellated Electromagnetic Space Structures for the Exploration of Reconfigurable, Adaptive Environments,’ says MIT Media Lab.
‘Each TESSERAE structure is made from a set of tiles. These tiles are tuned to autonomously self-assemble into a particular geometry – in our initial prototypes, we have focused on the buckminsterfullerene (20 hexagonal tiles, 12 pentagonal tiles).’
TESSERAE aims to function as multi-use, low-cost, orbiting modules that supply a critical Space infrastructure for the next generation of zero-gravity habitats, science labs and staging areas for on-surface exploration.
MIT Media Lab describes that ‘each tile at minimum includes a rigid outer shell, responsive sensing and control code for bonding diagnosis, electro-permanent magnets for dynamically controllable bonding actuation, and an onboard power harvesting and power management system.
‘Habitat-scale TESSERAE tiles will also include clamping and sealing for pressurisation. Tiles are released in microgravity testing environments to quasi-stochastically self-assemble.’
The concept focuses on supporting lunar and Mars operations, with dual-use orbit and surface capability:
- Tiles are packed flat and condensed for launch.
- Tiles are released after orbit insertion to quasi-stochastically self-assemble into the target geometry, while floating in microgravity.
- Once assembled, the structure can be reconfigured on demand (e.g., where a berthing port tile was needed yesterday, a cupola tile can be replaced tomorrow).
- Tiles can be disassembled entirely, packed flat again in an entry, descent and landing vehicle, and then deployed and snap-assembled with astronaut assists on the lunar or Martian surface.
Mirror, mirror…
Meanwhile, already out in space, the 2021-launched James Webb Space Telescope (JWST) is billed as ‘an unprecedented scientific instrument that will see further into the Universe than anything else ever built’.
Its light-collecting capability represents an unprecedented sensitivity and promises to enable a step-change in observing and analysing the Universe. It is tuned to detect infrared light which the Hubble Space Telescope (HST) is unable to.
The rocket, launched in 2021, sent the telescope about 1.6mln km from Earth. Unlike the HST, which has been orbiting the Earth since 1990, the JWST will orbit the Sun 1mln km from Earth.
It is fully robotic and has around 570 mirror optical surfaces. Almost half of the mirrors are installed within its mid-infrared instrument (MIRI).
Paul Shore, Director at Cranfield University, UK, spin-out Loxham Precision Limited and Head of Engineering at the National Physical Laboratory, says, ‘Cranfield developed the manufacturing capability and produced all the MIRI spectrometer mirrors.
‘This amounted to 132 flight mirrors on 24 substrate components, together with a full set of flight spares. The development of the JWST MIRI optics, from initial samples through to the delivery, was performed by the Cranfield team within a three-year period.’
The telescope should see light coming from the very first stars to shine in the Universe. The pioneer stars are thought to have switched on about 100-200 million years ago after the Big Bang, or a little over 13.5 billion years ago.
JWST will be picking out groupings of these stars. They are so far away that their light, despite moving at 300,000km/s, will have taken billions of years to travel the cosmos.
It should be possible for JWST to detect a faint glow from the moment when the darkness ended and those first stars flickered into life, and this could help understand the origins of everything we see around us and how planets form.
NASA has recently released landscape images of ‘mountains’ and ‘valleys’ speckled with glittering stars that were actually at the edge of a nearby, young, star-forming region called NGC 3324 in the Carina Nebula, captured in infrared light by the telescope. This image reveals for the first time previously invisible areas of star birth.
The telescope has also already captured the first clear evidence of carbon dioxide in the atmosphere of a planet outside the solar system. This observation of a gas giant planet orbiting a Sun-like star 700 light-years away provides important insights into the composition and formation of the planet. The finding suggests the instrument may be able to detect and measure carbon dioxide in the thinner atmospheres of smaller rocky planets.
Crest of a wave
Speaking of telescopes, the Universities of Sheffield and Warwick, UK, have also been working on a collaborative project to develop a new telescope, made up of two identical arrays, on La Palma in the Canary Islands and in Australia. The aim is to track down sources of gravitational waves for the first time.
Long hypothesised as a by-product of the collision and merger of cosmic behemoths such as neutron stars and black holes, gravitational waves were finally detected directly by LIGO (Laser Interferometry Gravitational-wave Observatory) in 2015.
The new Gravitational-wave Optical Transient Observer (GOTO) will be deployed on two opposite sides of the Earth to scour the skies for optical clues about the origin of these violent cosmic events, which create ripples, or waves, in the fabric of Space itself.
Martin Dyer, a Leverhulme Postdoctoral Researcher at the University of Sheffield, who works on GOTO, says, ‘Einstein predicted gravitational waves in his theory of relativity over 100 years ago, but we are just beginning to unravel what they can teach us about our Universe.’
‘If the gravitational wave observatories are the ears, picking up the sounds of the events, and the telescopes are the eyes, ready to view the event in all the wavelengths, then GOTO is the bit in the middle, telling the eyes where to look,’ adds Professor Danny Steeghs of the University of Warwick, GOTO’s Principal Investigator.
GOTO has received £3.2mln of funding from the UK Science and Technology Facilities Council (STFC) to deploy the full-scale facility, which will operate autonomously, patrolling the sky continuously but also focusing on particular events or regions of the sky in response to alerts of potential gravitational wave events.
Two telescope mount systems, each made up of eight individual 40cm telescopes, are now operational in La Palma. Combined, these 16 telescopes cover a very large field of view, with 800 million pixels across their digital sensors, enabling the array to sweep the visible sky every few nights.
Following the successful testing of this prototype system, the project is deploying a much expanded, second-generation instrument. The team is preparing a site at Australia’s Siding Spring Observatory, which will contain the same two-mount, 16-telescope system as the La Palma installation.
The plan is to have both sites operational this year to be ready for the next observing run of the LIGO/Virgo gravitational wave detectors in 2023.
Steeghs says, ‘The hope is to catch the event quickly, then follow it as it fades, and also to trigger an alert to other, bigger telescopes so they can all collect more information and we can build a really detailed picture of these astronomical phenomena.
‘It is a really dynamic and exciting time. In astronomy we are used to studying events which are millions of years old and aren’t going anywhere – this is a fast-paced, very different way of working where every minute counts.’
Inside the James Webb telescope
The James Webb Space Telescope (JWST) took 30 years and £7.5bln (US$10bln) to develop. It is seven times the size of the Hubble Space Telescope (HST), including all the associated equipment.
While the HST collects light using a main mirror of 2.4m, JWST has a primary mirror that is 6.5m in diameter.
The telescope has a cool side, which faces away from the Sun, and a hot side, which faces the Sun. Its tennis court-sized sunshield protects the telescope from external sources of light and heat, which ensures it can detect faint heat signals from very distant objects. It’s very important for its observing side to be very, very cold.
The lower part of Webb, where its five-layered sunshield is, faces the Sun. This is where its equipment that does not need to be cooled, like its solar panel, antennae, computer, gyroscopes and navigational jets, are housed.
Webb’s science instruments are housed behind the mirror, separated from the warm communications and control technology by the sunshield.