Quantum state of matter ‘tuned’ at will
An international team of researchers has discovered a quantum state of matter that can be ‘tuned’ at will – and it’s 10 times more tuneable than existing theories can explain. This level of manipulability opens enormous possibilities for next-generation nanotechnologies and quantum computing.
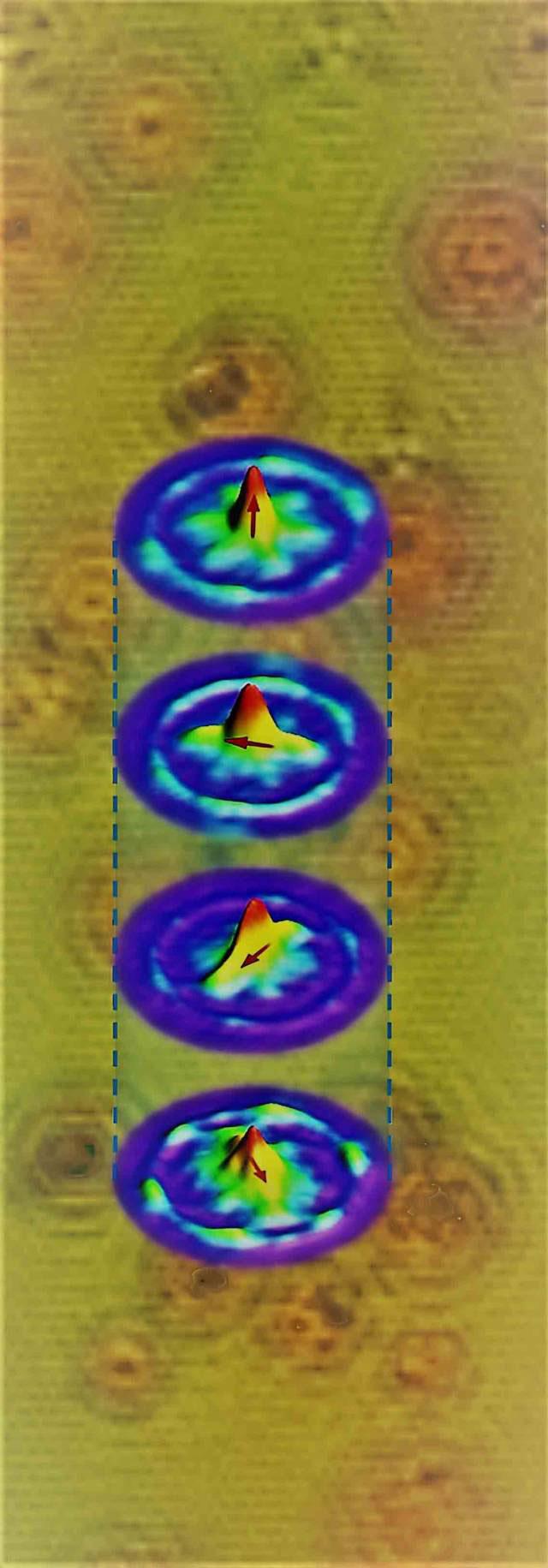
When the researchers turn an external magnetic field in different directions (indicated with arrows), they change the orientation of the linear electron flow above the kagome (six-fold) magnet, as seen in these electron wave interference patterns on the surface of a topological quantum kagome magnet. Each pattern is created by a particular direction of the external magnetic field applied on the sample.
© M. Z. Hasan, Jia-Xin Yin, Songtian Sonia Zhang/Princeton University‘We found a new control knob for the quantum topological world,’ says Zahid Hasan, the Eugene Higgins Professor of Physics at Princeton University, USA. ‘We expect this is tip of the iceberg. There will be a new subfield of materials or physics grown out of this. This would be a fantastic playground for nanoscale engineering.’
Hasan and his colleagues are calling their discovery a ‘novel’ quantum state of matter because it is not explained by existing theories of material properties.
Hasan’s interest in operating beyond the edges of known physics is what attracted Jia-Xin Yin, a postdoctoral research associate, to his lab.
‘When I talked to Professor Hasan, he told me something very interesting,’ Yin said. ‘He’s searching for new phases of matter. The question is undefined. What we need to do is search for the question rather than the answer.’
The classical phases of matter — solids, liquids and gases — arise from interactions between atoms or molecules. In a quantum phase of matter, the interactions take place between electrons, and are much more complex.
Hasan has been working in the ground-breaking subfield of topological materials, an area of condensed matter physics, where his team discovered topological quantum magnets a few years ago. In the current research, he and his colleagues ‘found a strange quantum effect on the new type of topological magnet that we can control at the quantum level,’ Hasan explains.
The key was looking not at individual particles but at the ways they interact with each other in the presence of a magnetic field. Some quantum particles, like humans, act differently alone than in a community. ‘You can study all the details of the fundamentals of the particles, but there’s no way to predict the culture, or the art, or the society, that will emerge when you put them together and they start to interact strongly with each other,’ he says.
To study this quantum ‘culture,’ he and his colleagues arranged atoms on the surface of crystals in many different patterns and watched what happened. They used various materials prepared by collaborating groups in China, Taiwan and Princeton. One particular arrangement, a six-fold honeycomb shape called a ‘kagome lattice’ for its resemblance to a Japanese basket-weaving pattern, led to something startling – but only when examined under a spectromicroscope in the presence of a strong magnetic field, equipment found in Hasan’s Laboratory for Topological Quantum Matter and Advanced Spectroscopy, located in the basement of Princeton’s Jadwin Hall.
All the known theories of physics predicted that the electrons would adhere to the six-fold underlying pattern, but instead, the electrons hovering above their atoms decided to march to their own drummer – in a straight line, with two-fold symmetry.
‘The electrons decided to reorient themselves,’ Hasan explains. ‘They ignored the lattice symmetry. They decided that to hop this way and that way, in one line, is easier than sideways. So this is the new frontier. Electrons can ignore the lattice and form their own society.’
This is a very rare effect, notes Hsieh. ‘I can count on one hand the number of quantum materials showing this behavior.’
The decoupling between the electrons and the arrangement of atoms was surprising enough, but then the researchers applied a magnetic field and discovered that they could turn that one line in any direction they chose. Without moving the crystal lattice, they could rotate the line of electrons just by controlling the magnetic field around them.
‘[We] noticed that when you apply the magnetic field, you can reorient their culture,’ Hasan says. ‘With human beings, you cannot change their culture so easily, but here it looks like she can control how to reorient the electrons’ many-body culture.’
The researchers can’t yet explain why.
Even more surprising than this decoupling – called anisotropy – is the scale of the effect, which is 100 times more than what theory predicts. Physicists characterise quantum-level magnetism with a term called the ‘g factor,’ which has no units. The g factor of an electron in a vacuum has been precisely calculated as very slightly more than two, but in this novel material, the researchers found an effective g factor of 210, when the electrons strongly interact with each other.
‘This gigantic and tunable quantum effect opens up the possibilities for new types of quantum technologies and nanotechnologies.’
The discovery was made using a two-story, multi-component instrument known as a scanning tunnelling spectromicroscope, operating in conjunction with a rotatable vector magnetic field capability, in the sub-basement of Jadwin Hall. The spectromicroscope has a resolution less than half the size of an atom, allowing it to scan individual atoms and detect details of their electrons while measuring the electrons’ energy and spin distribution. The instrument is cooled to near absolute zero and decoupled from the floor and the ceiling to prevent even atom-sized vibrations.