Graphene and nafion transistors work like synapses in the brain
The synaptic transistors have been developed by scientists at The University of Texas at Austin, USA, and can interact with living cells and tissue.
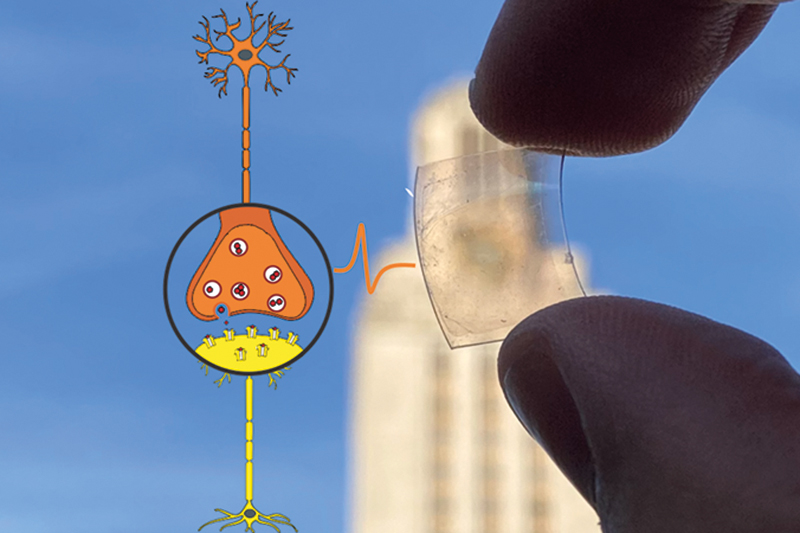
In this collaborative study, the thin, flexible graphene combines with nafion, a polymer membrane, to create biocompatible transistors known as BLASTs, which are non-toxic and work like the synapses in the brain.
With further work, the BLASTs could provide ideal materials for developing neuromorphic devices that mimic the processing capabilities of the brain, say researchers at the University’s Cockrell School of Engineering.
Graphene’s 2D structure is ultrasensitive to nafion, acting as a ‘gate’ material and delivering ‘synaptic pulses’. The researchers note graphene also offers faster switching speed and lower energy requirements.
Both materials are also biocompatible, flexible, soft and transparent. This is an important requirement for the potential future integration of artificial synapses with biological ones, explains Dmitry Kireev, a Post-Doctoral Researcher, who co-led the project. Most modern neuromorphic devices are rigid and inflexible and therefore incompatible with the brain.
'We are approaching learning from the brain and reproducing its functionality, both electrically and mechanically,' he explains.
'The latter is essential when we want to build systems that are not stand-alone ‘computing chips’ but smart decision-making elements for the future implants or the brain prosthesis elements.'
Typically, a classic transistor displays two states – on and off. A voltage is applied from a third terminal (the gate) to switch between the two states and it is the ratio between the two and the switch speed that defines the transistor’s quality.
Kireev says, 'Imagine that between ‘off’ and ‘on’ there are a dozen, hundreds or thousands of clearly defined and addressable levels. This is what we have in the biological neuron and what is reproduced via neuromorphic devices.'
According to the scientists, the BLAST produces the same number of contacts as neuromorphic devices – a single channel and single gate – but with multiple conduction levels or memory states.
'Since there are now multiple levels, it is essential that they do not degrade over time and that we can switch between these memory states with the least amount of energy,' says Kireev.
The BLASTs consume 50aJ/µm2 when they switch between the memory states and this is '20 times lower than the energy consumed by a biological synapse', claims Kireev.
He adds that the material can mimic 'brain-like parallel computing, with multiple memory states in a single device that can be reconfigured dynamically to perform certain computer tasks, such as image or pattern recognition'.
Although the work is still at the research and development stage, in the future, the transistor’s functionality could be applied to online learning, dynamic neuromorphic applications, which include visual or chemical sensing with live recognition.
'We have built small arrays of BLASTs, and we have also reported on even more peculiar neuromorphic properties such as the metaplastic feature, which helps in performing neuromorphic tasks such as image classification,' Kireev adds.
However, although 'these characteristics can be most immediately applied to a neural network accelerator', he estimates that it might take as long as 30 years before the devices are coupled with the brain and possibly used for implants, sensors or brain prosthetics.
Before the scientists can directly integrate the transistors with biological neural networks, they will first need to 'substantially reduce the dimensions of the neuromorphic BLASTs to a few µm2.'
Further work is also required to ensure that the transistors do not lose any performance quality and energy requirements from miniaturisation. The team may also need to re-engineer the transistor’s support structures and embed them in soft or flexible shells. If direct grant funding is available, they may integrate the BLASTs with the neuronal cells in vitro, possibly with organoids.