Going the distance with assistive technology
Assistive technology can have a transformative impact on people’s everyday lives. Andrea Gaini looks at some of the advances that are propelling this field.
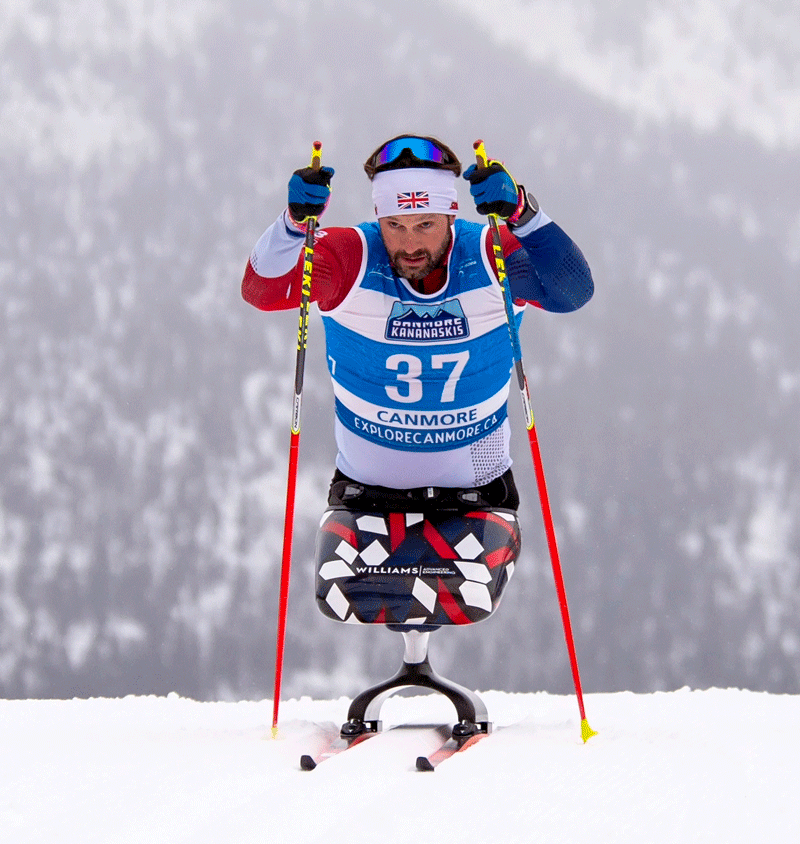
Assistive technology (AT) is defined as any item, piece of equipment, software programme, or product system used to increase, maintain, or improve the functional capabilities of persons with disabilities.
This definition from the UK Assistive Technology Industry Association may include technologies that have an electronic component, but also more broadly, any tool that is intended to replace a lost function.
Paul Doyle, an Assistive Technology Consultant since the 1990s, explains that one of the key advances in AT is the development of new materials.
‘Materials have certainly changed how assistive technologies have evolved over the years. If you think about some of the first prosthetic limbs, initially, they were made out of wood.
‘And over the years, those limbs have been fashioned out of newer and more modern materials. In the Second World War, for example, a lot of prosthetic lower limbs were fashioned out of tin and metal because they were lightweight and easy to produce.
‘More recently, limbs have become manufactured from high-tech alloys, lightweight alloys, and, even more recently, some of the more exotic materials, like carbon fibre.’
For improved fit, Doyle notes that research is now focused on snug, tight-fitting sockets to reduce the chances of slippage. ‘The challenge for the materials community is to develop limbs that can work well with osseointegration. So, effectively driving the anchoring point into the residual bone.’
Full throttle
Advanced materials, in particular, have paved the way for more robust, comfortable, and occasionally cheaper, AT products, claims Professor Andrew Parsons of the Department of Chemistry at the University of York, UK.
He points out that advances in nanotechnology and electrochemistry, for example, now allow metal to be woven into textiles, enabling electrical connectivity in the form of sensors or electronics to be embedded into a textile for health monitoring.
‘Also, developing biomaterials that reside in a biological environment opens up possibilities for regenerative medicine, with applications including eye implants.’
Parsons continues, ‘New materials have also found applications in prosthetics, orthotics and vision-related AT. Composite materials, such as glass- or carbon-fibre, allow for prosthetic and orthotic applications that are lightweight and can have a more dynamic response than traditional materials.
‘This includes J-shaped running blades used by amputee athletes, which are made of strong and lightweight carbon fibre. Also, although racing wheelchairs are commonly made from aluminium, an increasing number incorporate carbon fibre, which can make the chair lighter and more stable.’
He explains how highly flexible materials are also garnering interest for use in soft robots and exoskeletons, reducing actuation compared to more rigid exoskeleton suits. Exoskeletons, he says, are one of the most promising technologies in the field of AT.
‘Modern exoskeletons are devices that assist an individual to perform movements. Some exoskeletons are designed to help individuals perform rehabilitation after trauma or injury with the goal of recovering full mobility,’ explains Tommaso Lenzi, a Mechanical Engineering Assistant Professor at the University of Utah, USA. ‘Other exoskeletons are designed to assist an individual’s movements. These can help decrease an individual’s effort or increase their performance.’
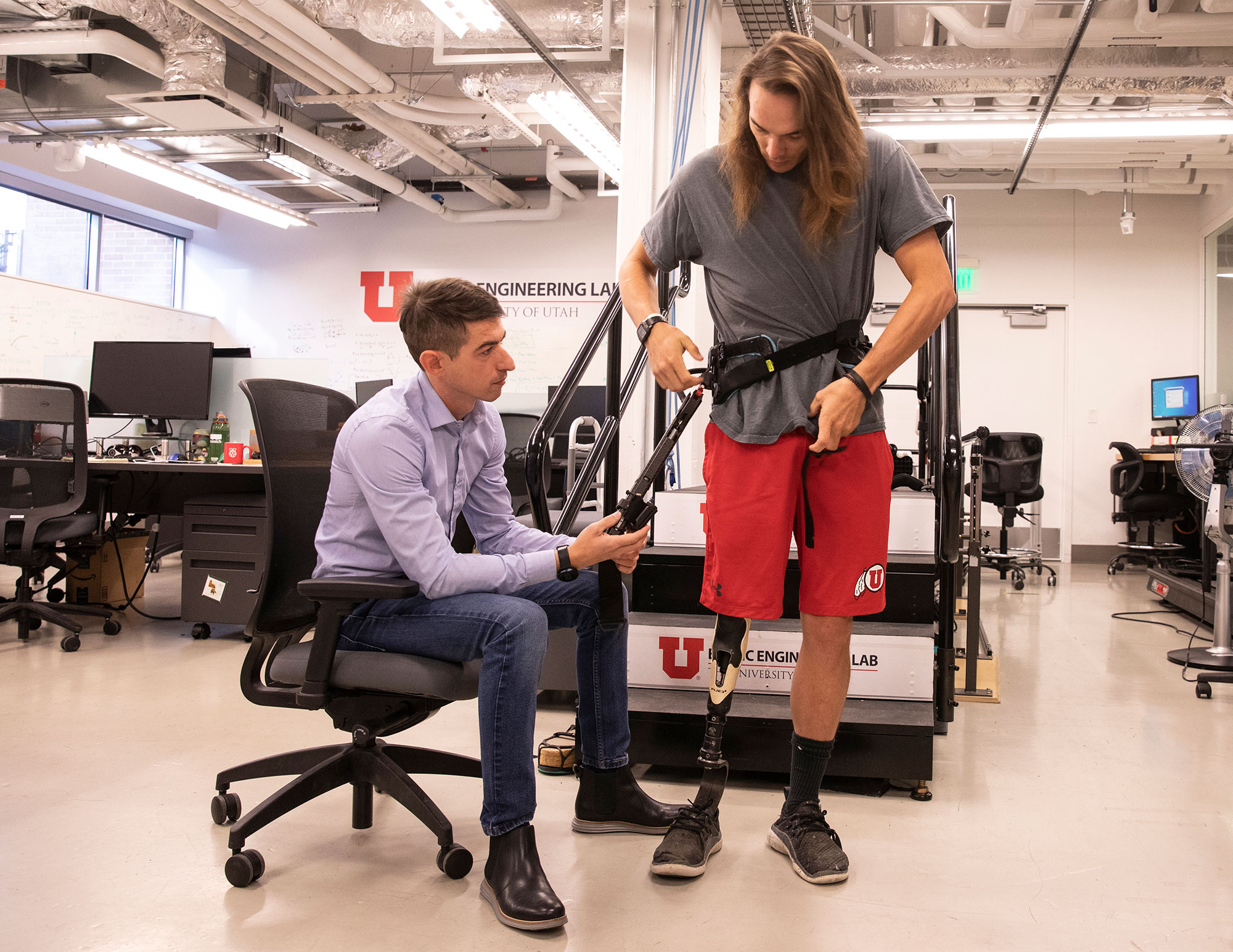
Lenzi describes how ‘exoskeletons are typically realised using electro-mechanical actuators. Many devices incorporate high performance electric motors with custom mechanisms. These can be incorporated in rigid frames or into soft suits. A few devices use pneumatic actuators’.
Did you know?
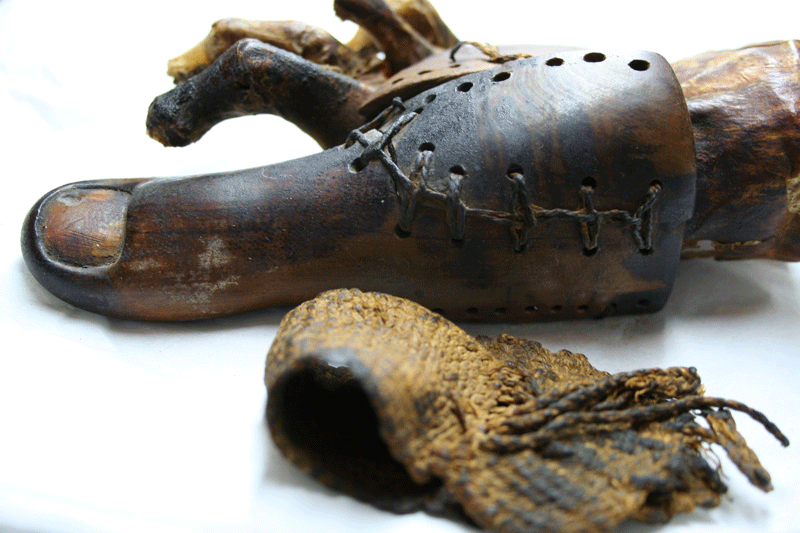
In 2012, the University of Manchester, UK, identified two wooden toes created by the Egyptians as possibly the world’s first example of assistive technology.
The Greville Chester toe, now at the British Museum, dates back to before 600BC and was made of cartonnage, an ancient type of papier maché using a mixture of linen, animal glue and tinted plaster. The other discovery is the wood and leather Cairo toe, dating back to between 950 and 710BC, found on a female mummy near Luxor, Egypt.
The researchers propose that the toes could have been developed as a way for the user to wear traditional Egyptian sandals, which would otherwise be difficult due to the increased pressure that a missing toe would put under the foot.
Experimenting with exoskeletons
Lenzi is part of a team that has developed an experimental exoskeleton made from aerospace-grade aluminium, steel and composite materials. The technology aims to allow users to walk with less effort thanks to a series of motors, microprocessors and advanced algorithms.
‘Whether it is a rehabilitation device or an assistive device, the exoskeleton applies forces and torques to the user’s limbs during movements. For example, some exoskeletons push or pull on the shank, thigh, or pelvis of an individual, supplementing the forces and torques generated by the user’s muscles,’ he says.
The research team’s brief was a device light enough that it was not a burden for someone to wear, yet provides high levels of assistive torque up to 40Nm.
The attachment points – the interfaces – that connect the exoskeleton to the user need to be stiff to transmit assistive forces and torques, yet comfortable and well-fitting so that the technology could be worn for long periods of time. The control algorithms need to adapt to different individuals, while still providing precise magnitude and timing of assistance.
To do so, the team has analysed gait biomechanics – the manner of walking – which reveals what forces are applied by the muscles to the limbs during movement. Then simulations predict the performance of an exoskeleton based on the gait biomechanics.
‘The result of the simulations is a virtual exoskeleton design that has an optimal function. We then used this virtual exoskeleton concept to guide the actual design of the exoskeleton in which we select lightweight, high-performance components to develop a prototype,’ he continues.
To compensate for the amputee’s overexertion of their residual-limb and intact-limb muscles, the exoskeleton provides assistive forces and torques to the thigh and pelvis while walking.
‘Fundamentally it connects to the residual limb, above the amputation level, and rhythmically pushes and pulls the leg during gait, so that the muscles in the residual limb can relax. In other words, the exoskeleton helps the user swing their leg forward in the air and pull their upper body forward over their leg while it is contact with the ground,’ Lenzi says.
The microprocessors developed in this study read the sensors embedded in the exoskeleton to measure how the user moves their legs during ambulation. Then, based on these movements, it determines the optimal action of the motors so that the exoskeleton can effectively assist the user’s gait. For example, the exoskeleton pushes the trailing leg as it swings forward during walking. The microprocessor performs this 1,000 times a second so that the assistance is always in sync with the user.
The microprocessor also communicates via Wi-Fi to a tablet or computer so that a therapist can monitor how the exoskeleton functions and make changes as necessary for each user.
Lenzi adds, ‘The transmission mechanism is mainly made of anodised 7075 aluminium with shafts made from 440°C hardened stainless steel. This mechanism is housed in a carbon fibre frame and, with the electric motor, makes the actuation unit. The actuation unit is attached to the pelvis and thigh through composite 3D-printed components and textile wraps.
‘We use electric batteries (lithium-polymer) to power the actuator and various embedded electronics to run the control routines, read sensor data, and transmit the data wirelessly to our control interfaces.’
To prepare these materials for use in the exoskeleton, Lenzi says that the aluminium and steel components are machined using rapid prototyping services. Some smaller parts also require wire electrical discharge machining or broaching techniques to create certain geometries. The steel parts are hardened, bead blasted, or finished as appropriate while aluminium parts are anodised.
The exoskeleton uses a combination of artificial intelligence (AI) and machine learning combined with robot control techniques to synchronise the assistance provided by the exoskeleton with the user. ‘So, the AI is continually learning the motion of the user. The exoskeleton assistance is then provided based on the learned motion to help the user accomplish that movement,’ Lenzi shares.
He expects that AI and other forms of machine learning will be used to develop intuitive control systems that provide assistance as desired by the user. These systems could take advantage of a variety of sensors to gauge user intent by measuring electrical signals from muscles or nerves.
‘AI and machine learning may also be used in the design process to help identify good candidate design.’
Helping hand
Doyle foresees that 3D printing also has the potential to come into its own when used for AT, enabling highly personalised interfaces between the person and the prosthetic, as well as rapid production.
‘I've worked with prosthetics and orthotics where the measurement of a residual limb, a stump, is done but then the process can be so long, that by the time the socket and the limb is delivered to the end-user, their physical stump has changed, has atrophied or grown, or the person has put weight on. And so, it can be quite a hit and miss process, interspersed with weeks and weeks of waiting. Whereas 3D printing can turn these things around in a matter of two or three days.’
Parsons continues, ‘Malleable materials such as nylon or epoxy resin have been designed that can be deposited, layer-upon-layer, in 3D printing. This offers an effective, relatively inexpensive and customised production of components, leading to more personalised AT products from wheelchairs to hearing aids to gloves for wheelchair racers.’
The Olympic dream
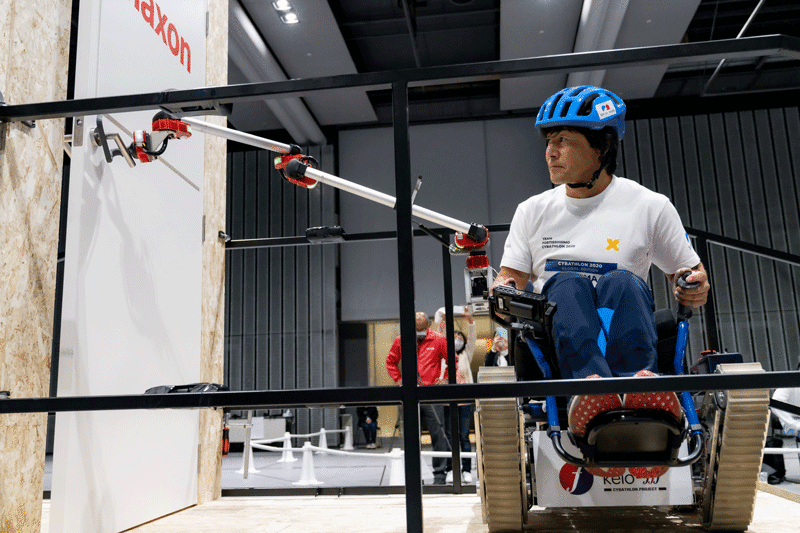
Since 2013, ETH Zürich, Switzerland, has been running the Cybathlon – the Olympics of Assistive Technologies.
The event challenges teams from all over the world to develop assistive technologies suitable for everyday use with and for people with physical disabilities.
More than 100 teams from over 30 countries have taken part in Cybathlon competitions in eight disciplines – an assistive robot race, vision assistance race, arm prosthesis race, brain-computer interface race, exoskeleton race, functional electrical simulation bike race, leg prosthesis race and a wheelchair race.
Observed and cheered on by thousands of spectators in the stadium and around the world, the development teams highlight the obstacles that people with disabilities face in their everyday lives and how technology can contribute to overcoming these barriers.
Teams consist of technology developers from universities, companies or NGOs, and a person with disabilities tackling various everyday tasks with the latest assistive technologies. The participants show how to tie shoelaces with a robotic arm prosthesis, balance on rocks with a prosthetic leg, or overcome uneven terrain with an exoskeleton.
The next games will take place from 25-27 October 2024.
Hitting the slopes
3D printing was used for Team GB’s equipment for the 2022 Paralympic Games in Beijing, China. The Sitskis, developed by Williams Advanced Engineering (WAE), has a main body of the fin and seat made from carbon fibre, while the interior and ski interface parts are made primarily of 3D-printed metals and plastics so that they can be complex, while still lightweight.
The skis were developed in a two-year programme. ‘We designed the concept in close collaboration with athletes, who provided feedback for every part of the journey, with a view to guaranteeing that the final product would be fully optimised for maximised performance,’ says Ollie Guild, Senior Product Designer at WAE.
Motorsport-derived techniques were a source of inspiration, including materials optimisation so that each part of the product is light yet durable and helps the athletes deliver energy straight into the snow.
‘The seat and fin structures have material placed only where strength is required, to keep the overall weight down, as well as being strategically located to ensure responsiveness on the snow,’ shares Guild.
‘Within each seat, the athlete has a moulded foam insert, inspired by the F1 driver seat fitting process, to make sure they are fully comfortable and ‘at one’ with their Sitski.
‘We also worked on the aerodynamics of the ‘fin’ below the seat, to make sure the Sitski glided as effectively as possible to prevent athlete fatigue, especially at high speed.’
WAE says they were in constant communication with the team during the construction, making sure the final product solved any concerns they had.
The Sitskis were tested through computer-aided engineering and digital materials testing, prior to validation of build at snow domes in the UK and Germany.
The location and positioning of the athlete in their seats above the skis are also key and so a number of different seat locations and angles were evaluated on balancing scales in the workshop. The rigs themselves were statically tested to understand their durability and wear throughout a race.
Throughout the summer season and during the pandemic when travel was restricted, the Sitskis were attached to ‘mountain boards’ used on roads in the UK to understand how the build, materials and athlete fit worked in real-life scenarios.
AT for everyday
Much like motorsport, where certain aspects of development are adapted for everyday cars and bikes, the work on assistive technologies for Paralympians is being taken into everyday products.
Guild explains that the Sitski has inspired the development of the DREAM wheelchair concept for young users.
WAE supported a review to provide recommendations on design, procurement and manufacturability to further aid the development of the wheelchair through its knowledge in the healthcare industry, developed through previous projects such as Babypod 2.0 and a Paralympic hand cycle, alongside its capability in product design and supply chain management.
He says, ‘The materials used ensure that weight and bulk is removed wherever possible and secondly [assure] the comfort of the user in regard to seating, which requires a high level of development and often custom additions.’
Doyle sums up, ‘Without these particular assistive technologies, people are unable to work and able to make a living for themselves. They are unable to read, learn, all these things that we take for granted.’