Characterising plastics waste
How characterisation of waste can enhance plastics recycling.
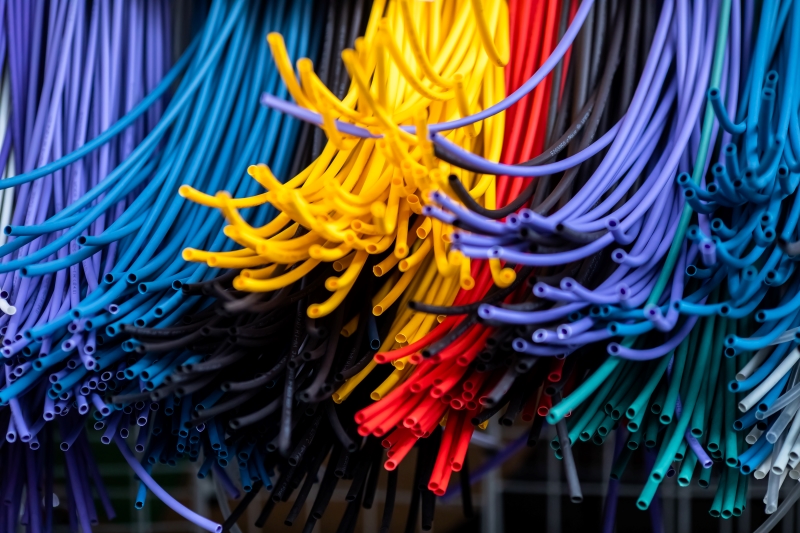
Plastic, a versatile and durable material, has infiltrated nearly every aspect of our daily lives, from packaging to electronics. With the relentless growth of plastic production and consumption in the modern world, the issue of plastic pollution has become an urgent global concern.
Plastics recycling is a pivotal solution against environmental degradation and waste management challenges. It involves collecting, processing and reusing plastic materials to divert them from landfills and incinerators. This not only conserves valuable resources but also reduces the energy and greenhouse gas emissions associated with the production of virgin material.
In recent years, advancements in recycling technologies, increased awareness about plastic pollution and legislative measures have propelled the plastics recycling industry forward. From household recycling bins to industrial recycling facilities, a concerted effort is being made to recover and repurpose plastics, transforming them into new products, thereby closing the loop in the lifecycle.
Breaking it down
Polyolefins are a class of thermoplastic polymers characterised by their simple structure, exceptional versatility and wide range of applications. These hydrocarbon-based polymers are known for their excellent chemical resistance, low cost and lightweight properties, making them fundamental materials in various industries. They consist of the following polymers:
Polypropylene (PP) for:
- Food packaging – including bottles, caps and containers, due to its excellent moisture resistance and durability
- Textiles, such as woven bags, ropes and geotextiles, thanks to its lightweight and strong properties
- Automotive interiors such as dashboards and bumpers for its resistance to heat and impact
High-density polyethylene (HDPE) for:
- Milk jugs, shampoo bottles and detergent containers due to its strength and chemical resistance
- Pipes for water and gas distribution due to its corrosion resistance and flexibility
- Outdoor furniture and playground equipment due to its durability.
Low-density polyethylene (LDPE) for:
- Plastic films for packaging such as plastic bags and shrink wrap
- Agricultural films for greenhouse coverings and mulching
- Squeeze bottles for condiments and lotions, due to its flexibility and easy dispensing.
Linear low-density polyethylene (LLDPE) for:
- Stretch-wrap films, providing excellent cling and protection for palletised goods
- Bin bags that are strong and puncture-resistant
- Industrial liners to protect and contain materials
Meanwhile, polyesters represent a versatile class of synthetic polymers known for their durability, flexibility and resistance to moisture and chemicals. Comprising compounds like polyethylene terephthalate and polybutylene terephthalate, polyesters find extensive use in textiles, packaging materials, engineering plastics and even biomedical applications due to their diverse and valuable properties.
Polymer recycling methods can be categorised as follows:
- Mechanical recycling – this is the most common method and involves collecting, sorting, cleaning and melting down plastics to create new products. In this process, plastics are mechanically shredded into small pieces, which are then melted and moulded to create new products. Mechanical recycling works well for plastics with consistent and homogeneous compositions and is commonly used for recycling plastic bottles, containers and automotive parts. It is a cost-effective method that helps reduce the environmental impact of plastics by reusing them in various applications.
- Chemical recycling – this is also known as feedstock recycling. It employs chemical processes like pyrolysis and depolymerisation to break down polymers into their original monomers or other valuable chemicals. This approach is suitable for plastics that are difficult to recycle mechanically, due to contamination, mixed compositions or complex structures. The products can then be used to produce new plastics or other chemicals, reducing the reliance on fossil fuels for raw materials.
- Bioplastics – these are derived from renewable sources like corn or sugarcane and represent an alternative to traditional plastics. Recycling bioplastics typically involves composting or converting them into biogas or bio-based materials.
Understanding the different types of polymer recycling is essential for designing effective waste management systems and promoting sustainable practices in the plastics industry. Each method has its advantages and limitations, depending on the type of plastic, its condition and the desired end-product, but collectively they contribute to reducing the environmental impact of plastic waste.
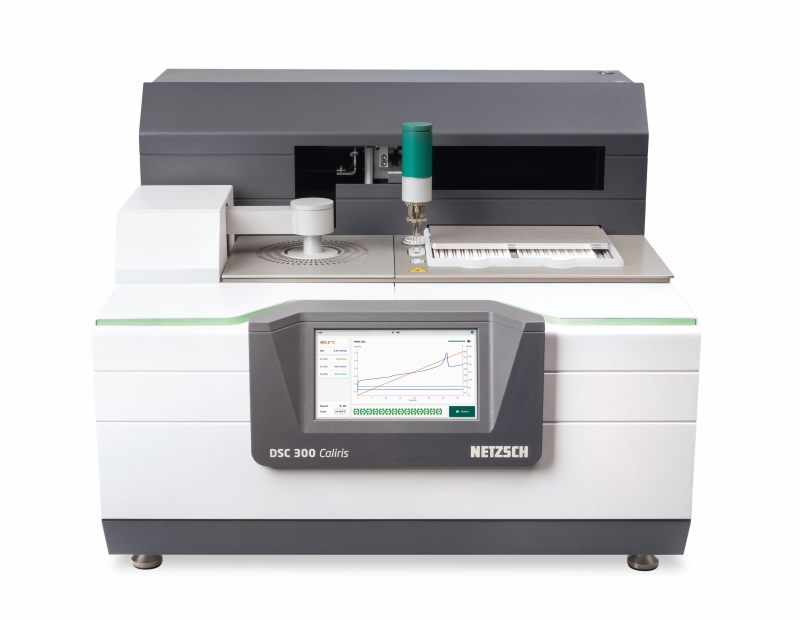
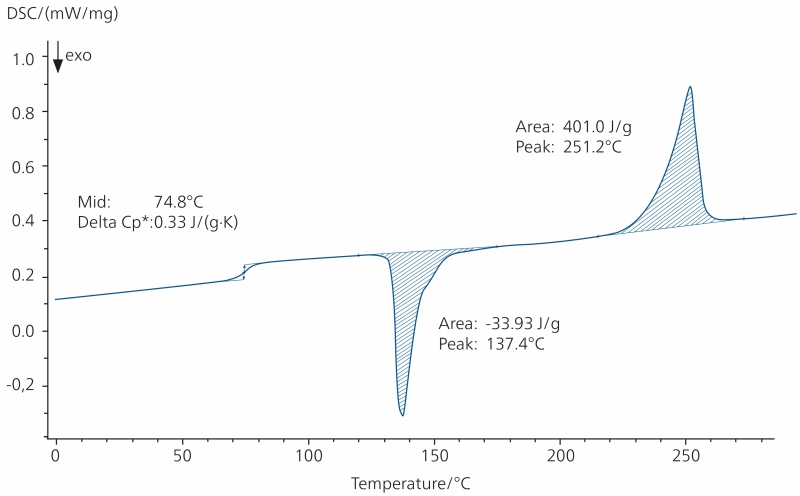
Turning to science
As the majority of plastics used in packaging are polyolefins, a combination of these materials can be found in our recycling streams. This poses a problem because PE and PP are immiscible and incompatible both in the molten and solid state. Nuclear Magnetic Resonance (NMR) and other fractionation methods have been successfully used to differentiate the content of PP into its fractions. However, these methods are expensive, time consuming and require high levels of expertise.
To identify plastics for recycling, characterisation tools such as Fourier Transform Infrared Spectrometry (FT-IR), a fast and easy laboratory technique, can be used. FT-IR provides a ‘chemical fingerprint spectrum’ to chemically identify one material from another for most polymers. However, it cannot differentiate between the different polyolefins such as HDPE, LDPE and LLDPE due to their similarity in chemical structures.
This is where thermal analysis and rheology techniques come in. These are critical techniques in the plastics recycling field, enhancing the efficiency and sustainability of the processes.
Thermal analysis involves studying how plastics behave under varying temperatures. This is essential for identifying the melting points, thermal stability and decomposition temperatures of different polymers. Understanding these characteristics helps to sort and process plastics based on their properties, ensuring that materials with similar melting points are recycled together. It also helps prevent plastics degradation during recycling, leading to higher-quality recycled products.
An alternative solution to FT-IR, which is proven to be suitable for mixed plastics waste and recycled polyolefin blends, is a Differential Scanning Calorimeter (DSC). In this instance, DSC uses the thermal fingerprint of a material, which is determined by the backbone structure, molecular weight, side groups and branching of the polymer. The significantly different melting temperatures of the materials can be used to identify the different components of a mixture, while their weight percentage is estimated based on the melting enthalpy.
Another thermal analysis technique commonly used in the plastics industry is thermogravimetric analysis (TGA). This method allows researchers and engineers to examine how different types of plastics react to increasing temperatures. It plays a crucial role in assessing the thermal stability and decomposition characteristics of polymers, making it an indispensable tool for optimising recycling processes and ensuring the highest quality of recycled materials.
By subjecting polymer samples to a controlled temperature ramp, TGA provides data on the changes in weight as the polymer undergoes thermal degradation. This information is vital for several key aspects of recycling:
- Material selection – TGA helps identify suitable polymers for recycling by determining their thermal properties. Understanding the degradation temperatures and decomposition profiles helps to select compatible materials for blending or co-processing.
- Process optimisation – TGA data helps determine the ideal processing temperatures and conditions to minimise polymer degradation while maximising resource recovery.
- Quality control – TGA monitors the thermal stability of recycled materials so that manufacturers can ensure they meet desired specifications. It can assist in determining homogeneity, including filler content, and distribution pre- and post-recycling.
- Feedstock analysis – TGA is also valuable for characterising the composition of mixed plastic waste streams. It helps identify and separate plastics based on their thermal behaviour, allowing for more efficient recycling.
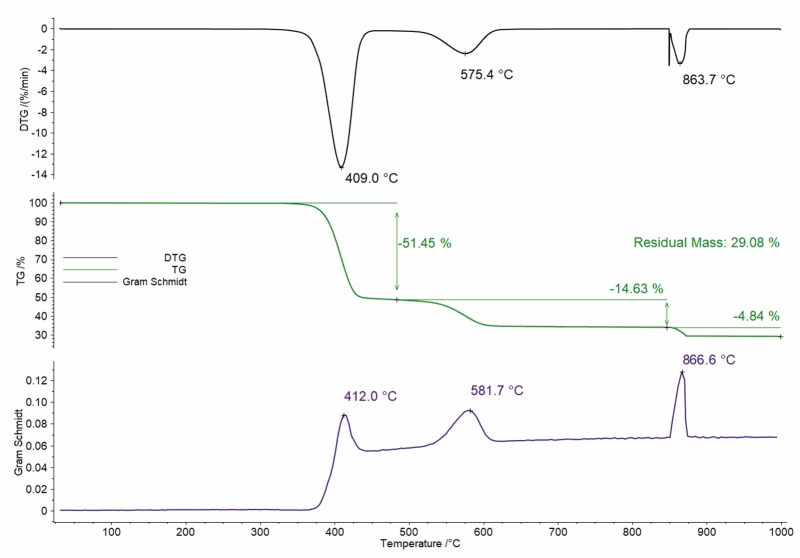
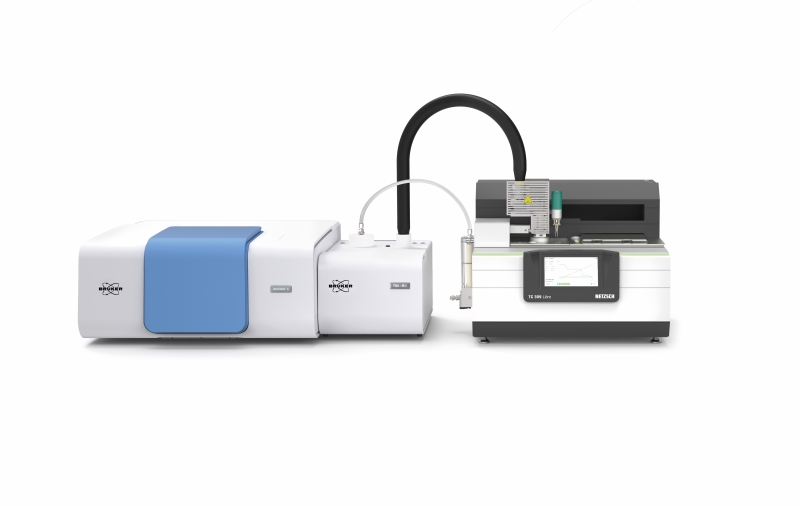
Rheology, on the other hand, studies plastics’ flow and deformation behaviour when subjected to mechanical forces or stresses. It can provide insight into crucial polymer properties such as molecular weight, molecular weight distribution and chain branching. These effect the polymer’s shear and extensional viscosity, which will in turn influence processing conditions or affect the final product performance. Rheology can therefore provide insights into how plastics can be processed efficiently during recycling, such as by optimising the extrusion or injection moulding processes.Rheological data can guide recycling equipment design and help achieve better quality control for recycled plastic products.
Understanding rheological behaviour is vital for polymer research to predict how material will behave and flow, to establish process development and develop innovative materials such as new biodegradable options. There is ongoing research in this area that heavily relies on rheology to enhance polymer recycling capabilities.
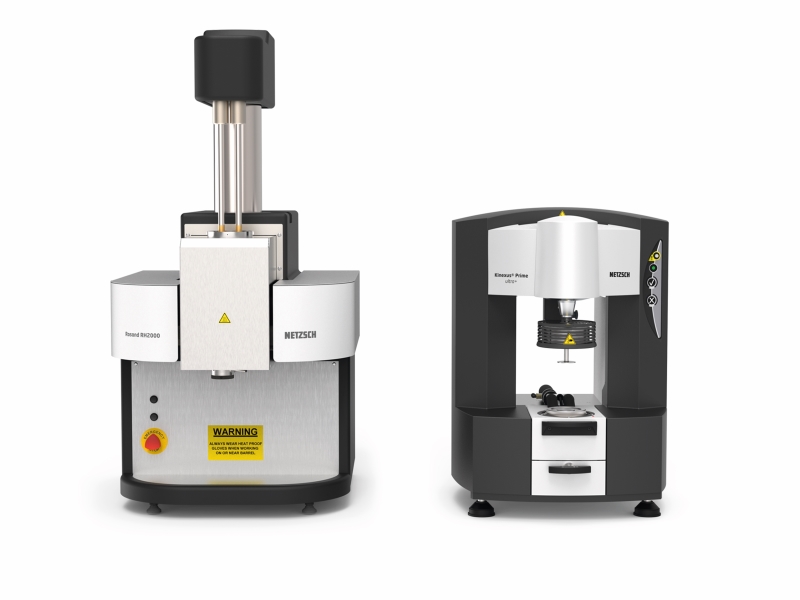
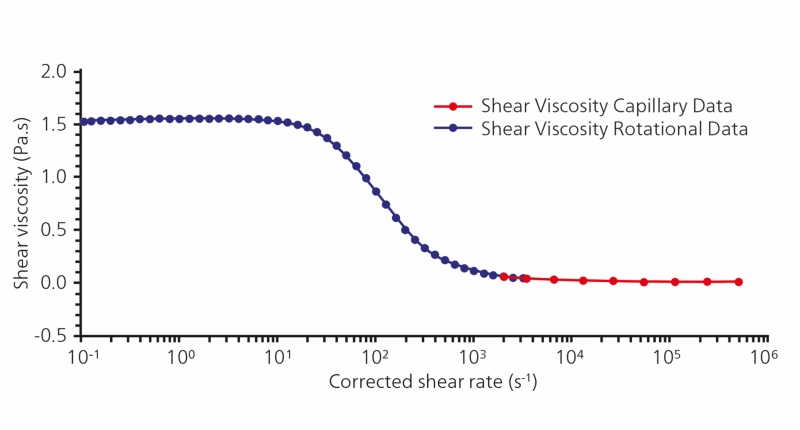
In the context of plastics recycling, the combination of thermal analysis and rheology enables the development of more sustainable and cost-effective recycling processes. It ensures that recycled plastics retain their desired properties, reducing waste and conserving resources. Ultimately, these analytical techniques are indispensable for advancing the circular economy and mitigating the environmental impact of plastics waste.
The future of polymer recycling holds promise as innovative technologies, increased awareness and sustainability imperatives drive progress. Advancements in sorting, chemical recycling and circular economy models will enable efficient plastics waste use, reducing environmental impact and conserving valuable resources.
Perhaps in a few years’ time we will see new ways of recycling, in addition to those mentioned above, because of the insights thermal analysis and rheology can provide.
If you are interested in research in this field, please contact Dr Shona Marsh at [email protected]